Mia E. Hoffman1,4, Bethany Sloane2, Anna Fragomeni3, Lisa K. Kenyon5, Sam W. Logan2, Katherine M. Steele1,4, Heather A. Feldner3,4
1University of Washington, Department of Mechanical Engineering, 2Oregon State University College of Public Health and Human Sciences, 3University of Washington, Department of Rehabilitation Medicine, 4University of Washington, Center for Research and Education on Accessible Technology and Experiences, 5Grand Valley State University, Department of Physical Therapy and Athletic Training
INTRODUCTION
Self-initiated mobility in any form is critical to the development of a child [1]. As toddlers explore their environment, they gain valuable experiences that contribute to their overall growth and well-being. For toddlers with mobility disabilities, powered mobility allows them the freedom to explore their environment, regardless of whether this intervention is short-term, while working on other mobility skill development, or long-term.
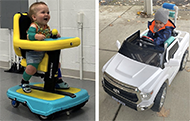
In the United States, there are currently two powered mobility options designed specifically for children 3 years of age or younger: the Permobil Explorer Mini (EM) and adapted ride-on cars (ROCs). The EM is a recently FDA-cleared pediatric mobility device for children ages 12-36 months. The device has adjustable seating, five speeds, and is controlled by a midline proportional joystick (see Figure 1-left). In contrast, the ROC is an off-the-shelf toy ride-on car where propulsion is modified by the family or community volunteers to enable activation with an accessible switch and provide structural supports (e.g., 5-point harness, headrest; see Figure 1-right). A Canadian study compared power mobility learning and skill progression in 46 children after use of 4 different powered mobility devices, including an ROC and three other devices not currently available in the United States. Results indicated that driving proficiency and learning increased across all devices [2]. However, due to the heterogeneity in young children's abilities and diagnoses, it can be difficult to compare device outcomes between participants, especially if device control (i.e. switch-adapted vs. joystick) or use frequency varies across devices. The same team that carried out the 6-month loan of four early powered mobility devices, also hosted powered mobility days where 74 children could trial up to four of the devices during a single 60-90 minute play session [3]. While both studies have demonstrated that a child's family's preference for a device varies and the device's configuration impacts a child's powered mobility skill, little is known about how children utilize different powered mobility devices in the home and community setting over time. Understanding how families integrate two different powered mobility devices into their life can help us select the proper device for families and inform the design of new powered mobility devices for children with disabilities under 3 years of age.
ID | Gender | GMFCS |
2 | F | V |
4 | F | V |
5 | F | V |
10 | F | V |
11 | F | II |
14 | F | IV |
15 | M | III |
17 | F | V |
In this paper, we quantified families' usage of the EM and the ROC. We compared the frequency of use, duration of play sessions, and environments in which the devices were used. We hypothesized that families would use the EM more than the ROC, drive longer distances, and take the device to more unique sites due to its smaller size and joystick control, which may contribute to easier in-home navigation and transport to other locations.
METHODS
We evaluated powered mobility use of 8 participants (1M:7F, Gross Motor Function Classification System (GMFCS) levels: II-V) and their families at 2 sites (University of Washington, Oregon State University) whose mobility devices were instrumented with a data logger. These participants were part of a larger randomized crossover clinical trial that provided 24 young children across 3 sites the EM and ROC for 8 weeks each [4]. The participants were between 12 and 36 months old and had been diagnosed with cerebral palsy (CP) or had high likelihood of CP diagnosis due to birth history and developmental status (Table 1).
A KJB Trail passive GPS data logger (KJB Security Products, Nashville, TN) was used to track the geospatial location of each participant measuring time, latitude, and longitude. First, we separated the data into distinct movement bouts. We defined a movement bout as two consecutive time points with a change in both latitude and longitude that occurred within 30 minutes of each other, and which occurred more than 30 minutes since the last bout. We removed GPS outliers by identifying coordinates that had a z-score greater than 3 for the GPS data for each bout collected for each child. Finally, we identified whether the device was being passively transported (e.g. driven in a car) or was being independently propelled using a velocity-based threshold.
Our primary outcomes were the number of movement bouts and duration of each movement bout. Additionally, we calculated the distance travelled and maximum distance the device travelled from the participant's home address using the Haversine formula with the geospatial coordinates . The Haversine formula is used to calculate the great-circle distance between two points on the surface of a sphere, and hence considers the curvature of the Earth's surface, unlike Euclidean distances. Finally, we analyzed maps to identify the number of unique locations they took the device and where they actively drove the device. All analysis was conducted using a custom-written Python script. A Wilcoxon signed rank test was utilized to compare usage between devices for each participant. All results are shown for time periods when the respective device was in use by the child (95.7% of all data), and not when the device was being transported in a vehicle.
RESULTS
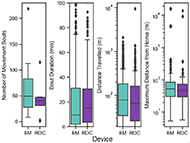
Participants used the EM more frequently than the ROC; however, this difference was not statistically significant (Figure 2). Children completed a total of 558 movement bouts with the EM compared to 325 with the ROC. The number of movement bouts for each child ranged from 8 to 219 with the EM and 1 to 115 with the ROC (Table 2).
While the participants completed more movement bouts with the EM, they spent longer periods driving the ROC. The average duration of a movement bout when a child was driving the EM was 23.16 min and 23.75 min with the ROC (Figure 2). Only one participant (P15) drove for a significantly longer time with the EM than the ROC.
Geospatial Analysis
Geospatial analyses revealed that all participants travelled a similar distance with both devices in each 8-week period, with distances driven during each bout ranging from 0 to 2.08 km (mean: 164 m) with the EM and 0 to 9.4 km (mean: 166 m) with the ROC (Figure 2).
ID | Bouts | Duration (min) | Distance Travelled (m) | Maximum Distance from Home (m) | ||||
EM | ROC | EM | ROC | EM | ROC | EM | ROC | |
2 | 8 | 1 | 11.6 | 2.7 | 56.0 | 172.4 | 6074.3 | 14029.8 |
4 | 28 | 3 | 12.5 | 4.9 | 97.6 | 53.5 | 187.0 | 372.9 |
5 | 14 | 48 | 17.4 | 20.3 | 73.9 | 74.4 | 16237.6 | 55.7 |
10 | 44 | 47 | 27.6 | 22.3 | 123.7 | 116.4 | 89.9 | 82.0 |
11 | 219 | 31 | 11.7 | 16.9 | 151.4 | 151.0 | 66.1 | 80.3 |
14 | 49 | 42 | 41.3 | 43.9 | 325.7 | 251.8 | 44.7 | 52.6 |
15 | 113 | 38 | 33.1 | 18.7 | 166.7 | 371.6 | 44.2 | 1268.7 |
17 | 83 | 115 | 32.3 | 22.6 | 168.6 | 133.9 | 38.0 | 37.6 |
Three participants (P4, P11, P15) drove their ROC to a location significantly further from their home than the EM (Figure 5). In contrast, only one participant (P5) actively drove their EM significantly further from home. P5 drove their EM exclusively in a different location than their home address. The maximum distance of the location from their home address that participants actively drove their device ranged from 5.2 m – 16.2 km with the EM and 5.7 m – 14.0 km with the ROC. We also investigated unique environments in which the devices were used. Only four families brought the device with them to another location and only three children used their EM or ROC at more than one location (Table 3). The ROC was transported to a unique location 5 times by 3 families in comparison to the EM that travelled to 2 unique sites with 2 families. However, the EM was actively driven at both sites that it was transported to, whereas the ROC was only driven at one unique location.
DISCUSSION
Participant | Number of Unique Sites Visited | Number of Unique Drive Sites | ||
EM | ROC | EM | ROC | |
2 | 2 | 3 | 2 | 1 |
4 | 2 | 1 | 2 | 1 |
5 | 1 | 1 | 1 | 1 |
10 | 1 | 2 | 1 | 1 |
11 | 1 | 1 | 1 | 1 |
14 | 1 | 1 | 1 | 1 |
15 | 1 | 3 | 1 | 2 |
17 | 1 | 1 | 1 | 1 |
In this work, we compared 8 children and their families' usage of an EM and an ROC over two months using metrics derived from geospatial analyses. We found that families' usage of both devices was comparable; however, more movement bouts occurred with the EM than the ROC. Participants in our study engaged in more driving bouts than reported in other studies. Logan et al. 2020 found that only 3 out of 14 families engaged in 14 or more sessions during the 3 months of use, whereas all but two of the families in our study used both devices 14 or more times over two months [5]. The duration of driving bouts in our study was comparable to those in other studies [5–7].
This is our second study to utilize geospatial analysis to characterize families' usage of early powered mobility devices. The distance that each participant drove with their device is similar to previous observations. In our prior work, children drove an average of 202 m outside with their ROC, and in this study, participants drove 166 m on average with their ROC and 164 m with their EM [8]. Participants in our current investigation typically used their ROC and EM near their home, with only two participants (P2, P5) using their EM at a distance greater than 1 km from their house and only two participants using their ROC (P2, P15) at a distance greater than 1 km from their house. This also aligns with our previous findings showing that the majority of device usage occurs within 300 m of a participant's home [8]. Fourout of eight participants travelled to another location with their EM or ROC. Families took their devices to parks, on road trips to nearby towns, to clinical visits, and to other residential addresses. The child may not have always driven at these locations, but the fact that it was in the car shows that the family views it as a mobility device, similar to a stroller, always ready to be used.
One limitation of this work is that geospatial data cannot always capture movement inside due to the building obstructing the signal. Hence, the usage of both devices may be even greater than we have captured. From previous research, we can hypothesize that the EM was used more frequently inside than the ROC due to its smaller footprint and 0° turning radius [5,9]. Future work could investigate this by using parent drive logs and a more complex data logger. Another limitation of this work is that we are not able to detect whether a child is driving the device themselves, or their parent or sibling is controlling it. Prior work has shown that parents often intervene to steer the ROC due to the difficulty a child might have manually doing so on their own [3,9]. We can hypothesize that parents also intervened in our current investigation to course correct with at least the ROC, and likely with the EM as well, but cannot confirm this due to limitations in our data logger.
CONCLUSION
This work shows that access to any early powered mobility device can be critical for a child's exploration of both their home environment and different locations in the community. Children had a higher number of movement bouts with the EM than the ROC, but usage of each device during an active driving bout was similar. Some families traveled with the device to different locations around their community, but most families primarily used it in the near vicinity of their homes. Provision of an early powered mobility device provides a family with an alternative mobility device for their child that allows them to determine their own path and increase independence, rather than being passively transported through their neighborhood (i.e., stroller, being carried). It is our hope that this work helps advocate for the need of children with disabilities to have access to any powered mobility device under the age of 3 years to promote mobility at similar times as their peers who are typically developing. We have shown that families will utilize the device if they have access to it. This work also shows that early powered mobility devices may need to be designed for different environments and distances, similar to adult mobility devices.
REFERENCES
[1] Feldner HA, Logan SW, Galloway JC. Why the time is right for a radical paradigm shift in early powered mobility: the role of powered mobility technology devices, policy and stakeholders. Disabil Rehabil Assist Technol 2015;11.
[2] Livingstone RW, Field DA. Exploring change in young children's power mobility skill following several months' experience. Disabil Rehabil Assist Technol 2020;0:1–10. https://doi.org/10.1080/17483107.2020.1847207.
[3] Livingstone RW, Bone J, Field DA. Beginning power mobility: An exploration of factors associated with child use of early power mobility devices and parent device preference. J Rehabil Assist Technol Eng 2020;7:2055668320926046. https://doi.org/10.1177/2055668320926046.
[4] Feldner HA, Logan SW, Kenyon LK. In the Driver's Seat: A Randomized, Crossover Clinical Trial Protocol Comparing Home and Community Use of the Permobil Explorer Mini and a Modified Ride-On Car by Children With Cerebral Palsy. Phys Ther 2022:pzac062. https://doi.org/10.1093/ptj/pzac062.
[5] Logan SW, Feldner HA, Bogart KR, Catena MA, Hospodar CM, Raja Vora J, et al. Perceived Barriers Before and After a 3-Month Period of Modified Ride-On Car Use. Pediatr Phys Ther 2020;32:243–8. https://doi.org/10.1097/PEP.0000000000000711.
[6] Logan SW, Huang H-H, Stahlin K, Galloway JC. Modified Ride-on Car for Mobility and Socialization: Single-Case Study of an Infant With Down Syndrome. Pediatr Phys Ther 2014;26:418–26. https://doi.org/10.1097/PEP.0000000000000070.
[7] Pritchard-Wiart L, Bragg E, Thompson-Hodgetts S. The Young Movers Project: A Case Series Describing Modified Toy Car Use as an Early Movement Option for Young Children With Mobility Limitations. Phys Occup Ther Pediatr 2019;39:598–613. https://doi.org/10.1080/01942638.2019.1585403.
[8] Hoffman ME, Steele KM, Froehlich JE, Winfree KN, Feldner HA. Off to the Park: A Geospatial Investigation of Ride-on Car Usage. Disabil Rehabil Assist Technol. Submitted.
[9] Logan SW, Feldner HA, Bogart KR, Catena MA, Hospodar CM, Raja Vora J, et al. Perceived Barriers of Modified Ride-On Car Use of Young Children With Disabilities: A Content Analysis. Pediatr Phys Ther 2020;32:129–35. https://doi.org/10.1097/PEP.0000000000000690.