Shoulder Joint Loading for Three Types of Lateral Wheelchair Transfers
Padmaja Kankipati, MS1, 2 , Alicia M. Koontz, PhD, RET1, 2 , Mike Turkovich, MS2
1 Human Engineering Research Laboratories, Highland Drive VA Medical Center, Pittsburgh, PA
2 Department of Rehabilitation Science and Technology, University of Pittsburgh, Pittsburgh, PA 15261
ABSTRACT
Wheelchair transfers have been associated with the high incidence of upper limb pain among persons with spinal cord injury. This study investigated shoulder kinetics for two types of lateral transfers incorporating the head-hips relation in comparison to the preferred method of transfer. Nine persons with paraplegia performed each transfer while motion analysis equipment recorded their upper body movements and force sensors recorded the forces applied by the hands. Average vertical shoulder forces in the trailing limb were lower using either head hips methods compared to the self-selected transfer. Keeping the leading arm close to the body during the head-hips motion minimized loading at the shoulder in the other directions. As a result this style of transfer may help to preserve upper limb function and maintain independence with transfers overtime. Future studies that include 3D kinematics of the trunk and upper extremities are needed to confirm these preliminary study findings.
KEYWORDS
Biomechanics, motion analysis, upper limb pain, spinal cord injury, activities of daily living.
BACKGROUND
People with lower limb dysfunction, like spinal cord injury (SCI), commonly have upper limb pain, due to their high reliance on their arms to perform activities of daily living such as wheelchair propulsion, pressure relief and transfers [1]. Being able to transfer independently is a key factor to achieving an optimal level of independence. Therefore any loss of upper limb function will severely affect overall functional mobility and independence.
There are different approaches that are used to perform transfer activities such as the lateral, front or back approach. The lateral transfer is the most common type of transfer since it is quick and requires less strength, and is essential for maintaining an independent lifestyle [2]. Allison et al. [3] described two general movement strategies used when performing lateral transfers: rotational strategy (head moves in an opposite direction to the pelvis) and translational strategy (head and pelvis move simultaneously in the same direction). When viewed from the sagittal plane, individuals performing the rotational strategy leaned forward during the transfer and those using the translational strategy kept their trunk more upright during the transfer. The rotational strategy is analogous to what clinical practice refers to as the ‘head-hips’ relation. It is often taught to patients with weak triceps and/or those with high levels of trunk involvement. Using a forward-flexed trunk position during transfers and pressure relief engages sternal pectoralis major and latissimus dorsi muscles [3]. This muscle substitution may help transfer the body weight between the leading arm (arm reaching to new surface) and trailing arm (arm behind during move to new location) with less loading of the glenohumeral joint thereby reducing the risk of rotator cuff impingement [3, 4].
While transferring, the shoulder is frequently placed in an impingement position which occurs when the arm is both internally rotated and abducted. This position is difficult to avoid when performing a level transfer without a transfer assist device (e.g., grab bar, trapeze, or transfer board). The purpose of this study was two-fold, 1) to compare shoulder joint kinetics for a level, lateral wheelchair transfer where the head-hips relation is used and the leading arm is abducted and away from the body (Head-Hips 1) and again with the leading arm close to the body and internally rotated (Head-Hips 2) and 2) to compare shoulder joint kinetics for each head-hips transfer to the participant’s own method of lateral transfer.
METHODS
Subjects:
After reading and providing informed consent, nine male subjects with SCI (Table 1), participated in this study. The inclusion criteria were: spinal cord injury C4 level or below that occurred over one year prior to the start of the study, able to independently transfer to/from a manual wheelchair without human assistance or assistive devices, over 18 years of age, and free from upper extremity pain that influenced their ability to transfer.
Subject |
Age |
Weight |
Height |
Duration of Injury |
Injury Level |
---|---|---|---|---|---|
1 |
38 |
78.93 |
1.73 |
13.25 |
T11-T12 |
2 |
33 |
63.96 |
1.73 |
11.5 |
T8 |
3 |
24 |
99.79 |
2.01 |
5.0 |
T8 |
4 |
47 |
58.97 |
1.83 |
26.5 |
T4 |
5 |
50 |
81.65 |
1.83 |
21.00 |
T6 |
6 |
35 |
124.74 |
1.75 |
12.25 |
L3 (incomplete) |
7 |
30 |
54.43 |
1.73 |
11.08 |
T9 |
8 |
20 |
72.12 |
1.55 |
16.00 |
T4 |
9 |
59 |
83.91 |
1.73 |
30.00 |
missing |
Mean |
37.33 |
79.83 |
1.76 |
16.29 |
T4-L3 |
Experimental Protocol:
Participants used their personal wheelchairs to transfer to and from a bench. For all transfers the wheelchair was positioned and secured at an angle of 30° from an adjustable height tub bench as shown in Figure
1. The bench was adjusted to be level with the subject’s wheelchair seat. The platform shown in Figure 1 contains two force plates (Bertec Corporation, Columbus, OH), one beneath the wheelchair and one beneath the tub bench. The wheelchair and bench were secured to the platform. A steel beam attached to a 6-component load cell (Model MC5 from AMTI, Watertown, MA) was positioned to simulate a wheelchair armrest. Reflective markers were placed on the subjects C7 and T3 vertebrae, right and left acromion processes, 3rd metacarpalphalangeal joints, radial and ulnar styloid processes, and lateral epicondyles. The coordinates of the markers were recorded based on a global reference frame using a six camera three-dimensional motion capture system (Vicon Peak, Lake Forest, CA). Several anthropometric measurements were recorded such as: axillary arm, wrist, fist and elbow circumference, upper arm and forearm length.
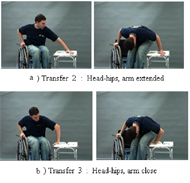
All transfers began with the left arm leading and moving the body from the wheelchair to the bench. For the first transfer, subjects were instructed to perform a lateral transfer as they normally would from their wheelchair to the adjacent level tub bench. For this transfer, they could place their left hand anywhere on the bench and right hand on the steel beam (height of wheelchair arm rest). The other two transfers were performed in random order. Prior to performing each of the transfer techniques, subjects were shown an instructional video on how to complete the transfer. For the Head Hips-1 (HH-1) transfer, subjects were instructed to place their left hand on the far target of the bench, right hand on the target on the force beam, and transfer leaning their trunk forward as far as possible while moving their buttocks toward the large target on the bench while moving their head in the opposite direction (figure 2a).The Head Hips-2 (HH-2) transfer required the same instructions except that the left hand was placed on the near target of the bench, with the left arm internally rotated (figure 2b). Subjects were allowed to practice before recording the transfer. Each transfer technique was performed three times and recorded at 60 Hz for the length of the transfer.
Data Analysis:
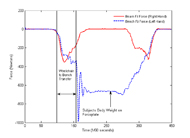
Kinetic, kinematic, and anthropometric data were entered into an inverse dynamic model to calculate the net shoulder joint force and moment. Kinetic and kinematic data were filtered with a 4th order zero-lag Butterworth filter (cut off frequency of 5 Hz and 7Hz respectively). The inverse dynamic model used was based on the general rigid-link segment model using a Newton-Euler method and a variable degree of freedom body co-ordinate system [5]. The beginning and the end of each transfer was determined from the vertical force data from the force plate under the tub bench and the force sensing beam. The increase of the forces followed by the decrease determines the lift phase of the transfer, where the arms are weight bearing, as shown in Figure 3. For this analysis, only data for the transfer from the wheelchair to tub bench were examined. Peak and average forces for the shoulder components and resultant force for both the leading and trailing arm were calculated for each transfer to the tub bench. Variables were computed using Matlab (Mathworks, Inc., Natwick, MA). Group means and standard deviations were determined.
Statistical Analysis:
Differences between the three types of transfers for each shoulder were evaluated using a repeated measure ANOVA or Friedman Test based on the distribution of variables. To determine differences between the leading and trailing arm a paired t-test or Wilcoxon Paired Signed Rank Test was used based on the distribution of variables. To test whether participant weight affected the shoulder joint forces a Pearson Correlation test was conducted. A significance of less than 0.05 was selected. The statistical tests were performed using SPSS statistical software (SPSS Inc., Chicago, IL).
RESULTS
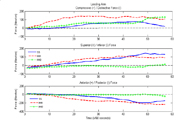
There was no significant correlation between participant weights and peak and average shoulder joint forces and peak shoulder moments. The forces from one representative subject for each of the three shoulder co-ordinate representations for the three types of transfers, for both the trailing and leading arm, are shown in Figure 3 and 4 respectively. This individual’s data showed a clear reduction in shoulder forces for the taught transfers compared to the self-selected style of transfer.
Differences between the three transfers
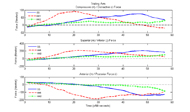
The compressive force in the leading arm was significantly lower (p = 0.008) for the self selected transfer compared to the HH -1 transfer (Table 2). The posterior and distractive forces were significantly smaller in the trailing arm for the self selected transfer (p = 0.011 and p = 0.008) when compared to the HH -1 transfer. The average superior/inferior forces in the trailing arm were found to be significantly lower for both the HH-1 (p = 0.021) and HH-2 (p=0.008) transfers when compared to the self selected transfer (Table 3).
Differences between leading and trailing arms
Peak compressive forces in the trailing arm were lower compared to the leading arm for the HH-1 and the HH-2 transfers (p = 0.028 and 0.008 respectively). Posterior forces in the leading arm were significantly higher than for the trailing arm for the HH-2 transfer (p=0.008). The distractive forces were significantly smaller in the leading arm compared to the trailing arm for the HH-1 & 2 transfers (p = 0.021 and 0.008 respectively). Resultant forces were significantly lower in the trailing arm compared to the leading arm for the HH-2 (p = 0.018) and self selected (p = 0.006) transfers. Average compressive/distractive forces for the HH-1 (p = 0.021) as well as the average anterior/posterior forces for the self selected transfer (p= 0.015) were significantly lower in the trailing arm.
Type of Transfer | Arm | Glenohumeral Joint to Acromion (N) |
Acromion to Glenohumeral Joint (N) |
Anterior to Posterior (N) |
Posterior to Anterior (N) |
Compressive (N) | Distractive (N) | |
---|---|---|---|---|---|---|---|---|
Self Selected |
Trailing Arm |
158.58 |
-22.33 |
-192.85 * |
28.47 |
55.46 |
|
|
Leading Arm |
168.48 |
-18.91 |
-260.68 |
22.47 |
68.85 * |
-25.17 |
||
Head Hips1 |
Trailing Arm |
109.40 |
-32.84 |
-255.27 * |
46.97 |
50.90 a |
|
|
Leading Arm |
140.27 |
-23.94 |
-258.44 |
20.98 |
107.94* a |
-24.24 a |
||
Head Hips2 |
Trailing Arm |
112.96 |
-18.38 |
-171.87 a |
35.73 |
39.65 a |
|
|
Leading Arm |
142.43 |
-23.41 |
-243.32 a |
23.06 |
83.35 a |
-4.21 a |
||
* between transfers, p<0.05 a between trailing and leading arm, p<0.05 |
Type of Transfer |
Arm |
Average |
Average Anterior/Posterior (N) |
Average |
Resultant Force |
---|---|---|---|---|---|
Self Selected |
Trailing Arm |
78.50 * |
-108.00 a |
0.41 |
263.69 a (30.05) |
Leading Arm |
80.42 |
-146.76 a |
22.34 |
335.62 a |
|
Head Hips1 |
Trailing Arm |
46.54 * |
-132.40 |
-6.26 a |
290.37 |
Leading Arm |
51.37 |
-137.02 |
40.77 a |
305.41 |
|
Head Hips2 |
Trailing Arm |
44.60 * |
-98.99 |
-6.15 |
252.06 a |
Leading Arm |
52.99 |
-140.54 |
37.42 |
321.44 a |
|
* between the three transfers, p<0.05 a between leading and trailing arm, p<0.05 |
DISCUSSION
The purpose of this study was twofold; to investigate shoulder joint loading for Head Hips-1&2 transfers and to compare the self selected transferring techniques to the taught transfers. The study had an added advantage of using experienced cohort of manual wheelchair users with SCI and testing transfers in a more natural, ‘real’ setting compared to other studies found in literature. This study found that transferring using either Head Hips technique reduced the overall force in the superior/inferior directions compared to the self selected transfer. One possible reason for this may be that our sample which consisted of high functioning individuals with paraplegia may have chosen a translational strategy for their self-selected transfer. When the trunk remains upright during the transfer, the shoulders are likely to bear more of the vertically-directed reaction forces versus when the trunk is flexed. When the trunk was flexed we saw a significant increase in the posteriorly-directed and medial-lateral force components (HH-1 transfer) compared to the self-selected transfer indicating that transferring with a more abducted leading arm, while reducing vertical loading at the shoulder, increased the demand on other muscles to laterally move the body. As a result, the HH-1 compared to HH-2, may be a more favorable head-hips transfer strategy because the shoulder vertical forces were significantly lower while the magnitudes of the other force components remained similar to those experienced during the self-selected transfer. However, with the HH-2 as with the self-selected transfer, more force was borne by the leading arm versus the trailing arm. Thus, consistent with Clinical Practice Guideline (6) recommendations, one should consider alternating which arm is leading and which arm is trailing with each transfer so as to evenly distribute the amount of cumulative loading experienced by each shoulder over the course of a day.
In general, we found, contrary to other studies, that forces in the leading arm were generally higher than the trailing arm for all the transfers. However, our study, is the first study to report shoulder kinetics during a transfer when a <simulated> armrest is used. Other studies either did not control for hand placement (if tested in a wheelchair) or the hand was positioned on a flat surface next to them
The findings of this study would be further enhanced if the degree of trunk flexion for each transfer was quantified. This information is necessary, for example, to determine how far the trunk needs to come forward in order to minimize the vertical loading at the shoulder. Likewise, future studies should also consider quantifying the amount of loading that is transferred to and supported by the lower extremities for each type of transfer.
CONCLUSION
Both Head Hips methods of transferring were found to reduce vertical loading at the shoulder when compared to the individual’s own method of transfer. Keeping the leading arm close to the body during the head-hips motion appears to minimize loading at the shoulder in the other directions. Therefore using this style of lateral transfer may reduce the risk of developing shoulder pain and impingement. Future studies that include 3D kinematics of the trunk and upper extremities are needed to confirm these preliminary study findings.
REFERENCES
- Davidoff G, et al. (1991). Compressive mononeuropathies of the upper extremity in chronic paraplegia. Paraplegia, 29, 17 24.
- Somers MF, Spinal cord injury functional rehabilitation, 2nd Ed., Prentice-Hall, Inc., Upper Saddle River, NJ 2001.
- D. Gagnon, S. Nadeau, D. Gravel, L. Noreau, C. Lariviere and D. Gagnon, Biomechanical analysis of a posterior transfer maneuver on a level surface in individuals with high and low-level spinal cord injuries. Clin Biomech 18, 319-331 (2003).
- Allison, GT and Singer KP, Assisted reach and transfers in individuals with tetraplegia: towards a solution. Spinal Cord 35, 217-222 (1997).
- Cooper, R.A., Boninger, M.L., Shimada, S.D., Lawrence, B.M. (1999) Glenohumeral joint kinematics and kinetics for three co-ordinate system representations during wheelchair propulsion. American Journal of Physical Medicine and Rehabilitation, 78(5), 435-446
- Preserving Upper Limb Function in Spinal Cord Injury: A Clinical Practice Guideline for Health-Care Professionals, in Spinal Cord Medicine, Clinical Practice Guideline. 2005, Consortium for Spinal Cord Medicine
ACKNOWLEDGEMENTS
Funding for this study was provided by the VA Rehabilitation Research R&D Services and the National Institute on Disability and Rehabilitation Research and the National Institute of Health, Center for Injury Research Control (VA-RR&D E3589V and B3079R NIDRR H133A011107, NIH R49/CCR310285-06).
Padmaja Kankipati, Human Engineering Research Labs, VA Pittsburgh Healthcare System, Pittsburgh, PA 15206 Phone: (412) 365-4829, Fax: (412) 365-4858, Email:kankipatip@herlpitt.org.