Wavelet Analysis of Blood Flow Oscillations to Assess Pressure Ulcer Risk in Older Adults
Yih-Kuen Jan, PT, PhD1 ; Bryan Struck, MD2 ; Robert Foreman, PhD3 ; Cyndy Robinson, MS, OT/L1
Departments of 1 Rehabilitation Sciences, 2 Geriatric Medicine, and 3 Physiology
University of Oklahoma Health Sciences Center, Oklahoma City, OK 73104
ABSTRACT
The objective of this study was to assess soft tissue viability and pressure ulcer risk in 20 older adults using wavelet analysis of blood flow oscillations (BFO). Sacral skin was heated to 42°C to induce a biphasic vasodilatory response. The results showed that increased age is associated with an increase in baseline blood flow and a decrease of vasodilatory function. The power of metabolic frequency is the most dominant frequency during nitric oxide mediated vasodilation, and the power of neurogenic frequency does not significantly contribute to the axon reflex mediated vasodilation. This study has shown the feasibility of using wavelet analysis of BFO to assess microvascular function for detection of the specific conditions which constitute the highest risk factors for pressure ulcers among older people.
KEYWORDS
Aging, blood flow oscillations, laser Doppler flowmetry, pressure ulcer, wavelet analysis.
BACKGROUND
Pressure ulcers significantly impact the quality of life and increase overall healthcare costs in elderly wheelchair users. The natural aging process may induce changes of structure and function of the cardiovascular system, thus increasing risk for pressure ulcers (1). Although aging-associated risk for pressure ulcer is not preventable, an effective risk assessment tool could help clinicians identify at-risk people to provide preventive interventions. For current clinical practice, a number of risk assessment tools (e.g. Braden, Norton, and Waterloo scales) have been proven to have good sensitivity to identify at-risk people; however, the specificity of these tools is not adequate to identify the highest risk people (2). To further identify people at the highest risk from a risk group identified by the Braden scale, we speculated that skin microvascular function may be a good venue for achieving this goal (3).
An important role of skin microcirculation is to regulate blood flow to meet the metabolic demands of local cells and to adjust skin temperature. Prolonged sitting and bedridden postures have been observed in older people due to loss of muscle strength, poor balance, and poor general health. A consequence of this prolonged contact of soft tissue with a support surface is the accumulation of body heat and an increase in skin temperature. The increased temperature raises the metabolic demands of local cells and tissues. The inability of skin microcirculation to remove excessive heat by increasing blood flow results in tissue ischemia, thus increasing risk for pressure ulcers (4).
RESEARCH QUESTION
In this study, we examined the aging effect on microvascular function and explored a new quantification method to study the contribution of each control mechanism to vasodilation by comparing time-series changes of power (amplitude) of each characteristic frequency. We also tested whether an increase in skin blood flow during the second peak is primarily mediated by the 0.01 Hz frequency.
METHOD
Twenty healthy older participants (10 aged 65-75 years old (6 male, 4 female) and 10 aged 75-85 years old (5 male, 5 female)) were recruited into the study. The demographic data of the 65-75 years group were as follows (values are mean ± standard deviation): age, 70.0±3.4 years; height, 1.74±0.15 m; and weight, 81.3±9.9 kg. The demographic data of the 75-85 years group were as follows: age, 78.2±2.0 years; height, 1.67±0.10 m; and weight, 70.0±10.4 kg. The exclusion criteria included the presence of pressure ulcers, diabetic mellitus, cardiopulmonary diseases, or use of any medications that may affect cardiopulmonary function. The study was approved by the University Institutional Review Board. Informed consent was obtained from each participant prior to any testing.
The laser Doppler flowmetry (LDF) (Vasamedics, Eden Prairie, MN) was used to measure skin blood flow. The analog output of the LDF device was sampled at 20 Hz . A heating probe (Vasamedics) was used to heat the skin to 42°C in 2 minutes. The heating probe (28mm diameter) has a central hole to accommodate the LDF probe (1mm diameter). This heating protocol was designed to differentiate axon reflex mediated vasodilation from nitric oxide mediated vasodilation (5). We proposed a Biphasic Thermal Index (BTI) to quantify the biphasic vasodilatory response. The BTI consists of three ratios, which are defined as ratio of the first peak to baseline blood flow, ratio of the nadir to baseline blood flow, and ratio of the second peak to baseline blood flow. The first value of the BTI relates to the sensory axon reflex function and the third value indicates endothelial nitric oxide function. The meaning of the second value remains to be elucidated (5).
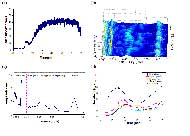
Graph 1: d Wavelet Analysis of Blood Flow Oscillations (Click for larger view)
Continuous wavelet transform was used to calculate the power within each characteristic frequency. Details of the wavelet analysis and quantification methods used have been described in our previous research studies (3, 6). Here, we provided an example of skin blood flow response to local heating and transformation of skin blood flow from the time domain to the time-frequency domain (Graph 1: (a), (b), and (c)). These five characteristic frequencies reflect the activity of metabolic endothelial related controls (0.0095-0.02 Hz), sympathetic neurogenic systems (0.02-0.05 Hz), vascular smooth muscle activities (0.05-0.15 Hz), breathing movements (0.15-0.4 Hz), and heart beats (0.4-2.0 Hz) (Graph 1(c)). Graph 1(a) shows skin blood flow response to rapid local heating during pre-heating (1-10 min), heating (11-60 min), and post-heating (61-70 min) periods. Continuous wavelet transforms of the blood flow signal in Graph 1(a) produce a scalogram (Graph 1(b)), in which time-frequency information on blood flow oscillations has been shown. By averaging wavelet coefficients over a 10 minute period, a wavelet-based power spectrum has been obtained and shown in Graph 1(c). If we perform the average during a one-minute period, then the time-series amplitudes of five characteristic frequencies could be obtained (Graph 1(d)). Data in this format allow for the examination of the relative activities of five frequencies. In our case, the method allows us to study the contribution of each control mechanism to the vasodilatory response.
The times to the first peak, nadir, and second peak of the biphasic blood flow response to local heating were identified as well as the corresponding blood flow value at each of the three levels. Nonparametric tests were used in this study. Friedman tests were used to examine the statistical difference among metabolic, neurogenic, and myogenic control mechanisms for the within-subject comparison. Wilcoxon signed rank tests were used for between-subject comparisons. The level of significance for post hoc multiple comparisons of repeated measures was adjusted to 0.017 based on the Bonferroni correction.
RESULTS
Skin blood flow and BFO values were calculated for the 65-75 years group first to establish the basis for the subsequent comparisons between 65-75 and 75-85 years groups. Mean skin blood flow of 10 older people shows a biphasic response to local heating in Figure 2. The first peak occurs at the 4th minute after heating (13.3±0.4 arbitrary units (au) or mLLD/min per 100g tissue), followed by a nadir at the 6th minute (10.9±0.4 au), and the second peak at the 40th minute (24.8±0.5 au) (Graph 2).
For the absolute amplitudes of characteristic frequencies, the Wilcoxon-Signed Ranks tests indicated that metabolic control is higher than both neurogenic and myogenic controls during all heating periods (p<.017). To compare the relative changes of three blood flow controls under local heating, the amplitude of each control was normalized to its baseline amplitude. The results showed that metabolic control is significantly higher than myogenic control during the 11-20 minutes of local heating protocol (i.e. the first ten minutes of local heating) (p<.017).
By averaging wavelet coefficients of each frequency in a one-minute period (method shown in Figure 1), the instantaneous activity of metabolic, neurogenic, myogenic, respiratory, and cardiac controls of the 65-75 years group were quantified (Graph 3). The result showed the metabolic frequency is the most dominant frequency during biphasic vasodilation. Other characteristic frequencies (neurogenic, myogenic, respiratory, and cardiac) also show an increasing trend but at a lesser degree. The metabolic frequency showed an early increase as compared with the increase in skin blood flow.
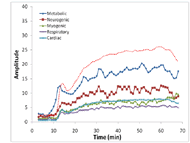
The skin blood flow (absolute and normalized values), Biphasic Thermal Index, and power within each characteristic frequency of the 75-85 years group were compared with data obtained from the 65-75 years group. The result indicated that the 75-85 years group has a higher baseline blood flow but a lower maximal blood flow as compared with the 65-75 years group (p<.05). For the Biphasic Thermal Index, the values were (3.7, 3.2, 6.7) for the 75-85 years group and is significantly different with the values of (5.5, 4.5, and 10.2) for the 65-75 years group (p<.017). The Wilcoxon-Signed Ranks tests indicated significant differences in all three characteristic frequencies during the first peak and second peak of biphasic vasodilation (p<.017).
DISCUSSION
In the present study, by using wavelet-based spectrum analysis of skin blood flow response to a fast heating protocol, we confirmed that the 0.01 Hz frequency (ranging from 0.008 Hz to 0.02 Hz) relates to the endothelial nitric oxide activity. To the best of our knowledge, this is the first study to analyze the biphasic blood flow response using wavelet analysis. The endothelial related frequency (0.01 Hz embedded in BFO) has garnered much attention due to its potential impact on the early detection of endothelial dysfunction and its related diseases (pressure ulcers in our case). If our analysis method has been validated, the non-invasive and easy to use LDF has great potential to become a clinical routine for identification of people at highest risk for pressure ulcers from a risk group identified by the Braden scale.
Our long-term goal is to develop non-invasive assessment tools for the detection of older people who are at the highest risk for pressure ulcers based on microvascular reactivity. This tool is to further classify the risk level among people identified as at-risk by the Braden scale. The noninvasive nature of monitoring blood flow control mechanisms (metabolic, neurogenic, and myogenic) provides a methodological advantage over traditional invasive or in vitro studies, in which maintenance of the integrity of the microcirculatory system is impossible. With the proposed wavelet-based spectrum analysis of LDF blood flow oscillations, clinicians may use the LDF to assess tissue viability and pressure ulcer risk in older people. By assessing the underlying mechanism responsible for the attenuated vasodilatory response, clinicians could deliver specific interventions (e.g. physical activity-based rehabilitation) to overcome this impairment to enhance soft tissue viability. Our results also support the use of the Biphasic Thermal Index to assess soft tissue viability in older people whose baseline blood flow is higher and maximal blood flow is lower.
Our results support the hypothesis that the 0.01 Hz (0.008-0.02 Hz) frequency embedded in blood flow oscillations is responsible for the increase of blood flow under a rapid local heating. Researchers have proven that the physiological mechanism responsible for vasodilation is endothelial nitric oxide. This finding indirectly supports that the 0.01Hz oscillatory component relates with endothelial nitric oxide. We demonstrated that older people have a lower first peak blood flow of the biphasic blood flow response to local heating. By averaging wavelet coefficients of each characteristic frequency in a one-minute period, we demonstrated that five blood flow controls have synergic regulation for enhancing blood flow. Specifically, this method has great potential to improve our understanding about the regulation of cutaneous microcirculation by simultaneous monitoring nitric oxide control and its interactions with other blood flow control mechanisms.
REFERENCES
- Marin J. (1995). Age-related changes in vascular responses: a review. Mechanisms of Ageing & Development. 79: 71-114.
- Moore ZEH and Cowman S (2008). Risk assessment tools for the prevention of pressure ulcers. Cochrane Database of Systematic Reviews. 3: CD006471.
- Jan YK, Brienza DM, and Geyer MJ (2005). Analysis of week-to-week variability in skin blood flow measurements using wavelet transforms. Clinical Physiology & Functional Imaging. 25: 253-62.
- Harder, Y., et al. (2007). Aging is associated with an increased susceptibility to ischaemic necrosis due to microvascular perfusion failure but not a reduction in ischaemic tolerance. Clinical Science. 112: 429-440.
- Minson CT, Berry LT, and Joyner MJ (2001). Nitric oxide and neurally mediated regulation of skin blood flow during local heating. Journal of Applied Physiology. 91: 1619-26.
- Geyer, M.J., Jan YK, Brienza DM, and Boninger ML (2004). Using wavelet analysis to characterize the thermoregulatory mechanisms of sacral skin blood flow. JRRD. 41: 797-806.
ACKNOWLEDGEMENTS
This work was supported by Mary E. Switzer Fellowship, National Institute on Disability and Rehabilitation Research, US Department of Education (grant no. H133F060025).
Author Contact Information:
Yih-Kuen Jan, PT, PhD, Department of Rehabilitation Sciences, University of Oklahoma Health Sciences Center, 801 NE 13th Street, Room 249, Oklahoma City, OK 73104, e-mail: yjan@ouhsc.edu.