Chao-Chen Lo1,3, Hsiao-Yu Lee2, Jia-Jin J. Chen3
1Shu-Zen Junior College of Medicine and Management1, Far East University2, National Cheng Kung University3
Tainan, Taiwan, ROC
INTRODUCTION
Stroke, a leading cause of hemiparesis, is usually exhibiting asymmetrical movement pattern [1]. One of the major goals of neurorehabilitation for stroke is to achieve better symmetrical movement. Such asymmetrical pattern could be found in bilateral movement such as gait performance or cycling movement [2, 3]. Prolonged immobilization can lead to severe deconditioning, such as muscle atrophy, loss of bone mass and reduced cardiopulmonary function. However, conducting passive range of motion to those critical illness could be a burden for caregivers and motor recovery is found to be limited. Passive cycling has been proven to be beneficial for ambulatory recovery [4]. Along with passive and active cycling, electrical stimulation could be used to facilitate muscle contraction and functional movement of paretic muscles [5]. It has been proposed that low-intensity of stimulation could better facilitate volitional contraction in isometric contraction [6]. There is still no agreement on the application of intensity level for electrical stimulation-assisted cycling and recently research has changed to investigate the stimulation effects on cortical activation, the origin of movement. Near infrared spectroscopy (NIRS) is a non-invasive method for cortical activation by measuring changes in oxyhemoglobin and deoxyhemoglobin. Therefore, the aim of our study is to investigate the effect of different intensity level of electrical stimulation assisted cycling on cortical activation evaluated by NIRS.
METHODS
Participants
Sixteen subjects, nine stroke patients and seven normal young subjects, were recruited from a tertiary medical center. The inclusion criterion for stroke was ischemic stroke causing hemiparesis and can maintain sitting position for over 30 minutes. Those with other neurological disease or orthopedic conditions in lower extremities were excluded. As for control group, seven normal subjects without stroke history were included. The study protocol was examined and approved by the Institutional Review Board of Kaohsiung Veterans General Hospital. The experimental procedures were informed to all subjects and written consents were signed.
Experimental procedures
Subjects were asked to relax during passive cycling tasks at a pace of 50 rpm which can be easily followed by the testing subject. During passive cycling with different intensity level, each task started with a 40-s rest of baseline and eight repetitions of a 20-s cycling period with a 40-s rest period in between. There are three different electrical stimulation intensity levels during passive cycling with a random order: (1) without electrical stimulation (NES), (2) with low-intensity electrical stimulation (LES) of 10 mA and (3) with high-intensity electrical stimulation (HES) of 30 mA. The stimulation was applied on affected quadriceps with biphasic pulses of 20 Hz and 300 µs at specific angles for better facilitation. Dead spots, transitions between hip flexion and extension, is calculate by tangent distances between hip joint and crank center. Then the stimulation angular range was set according to the dead spots [7].
NIRS measurement
Signals of hemodynamics, changes in oxyhemoglobin and deoxyhemoglobin, were measured using a continuous-wave NIRS, NIRScout (NIRx Medizintechnik GmbH, Germany). The system is equipped with wavelengths of 760 and 850 nm, 6.25 sampling rate. Seven sources and sixteen detectors were used to compose 28 channels with the concept of 10-20 system, covering premotor cortex (PMC), supplementary area (SMA), sensorimotor cortex (SMC), and secondary sensory cortex (S2) [8].
Data analysis
NIRS data were analyzed by an open-source software HomER [9]. The data were then filtered by a 3rd IIR Butterworth band-pass-filter between 0.0016 and 0.8 Hz to eliminate slow drifts and cardiac pulsation. The concentration changes of oxyhemoglobin of eight repetitions were averaged. According to neurovascular coupling, oxyhemoglobin increases and deoxyhemoglobin decreases slightly with the task. Therefore, concentration changes of oxyhemoglobin could be seen as an indicator of cortical activation. An optical brain image of oxyhemoglobin changes during cycling was constructed through HomER back-projection and tomographic algorithms [10].
The results, oxyhemoglobin changes of different regions (PMC, SMA, SMC, and S2) at three different intensity levels (HES, LES, NES) in normal and stroke patients, were statistically analyzed by multivariate analysis of variance (MANOVA, SPSS software). Wilcoxon signed rank test was then applied after significant multivariate effects were found. A value of p < 0.1 was set to be statistically significant.
RESULTS
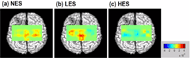
Significant multivariate effects were found in affected (p = 0.004) and unaffected S2 (p = 0.027). Wilcoxon signed rank tests then show significant differences between NES and LES (p = 0.018 for affected S2 and p = 0.017 for unaffected S2) together with between LES and HES (p = 0.058 for affected S2 and p = 0.050 for unaffected S2). Greater activation was found in affected S2 under LES (10.2±1.7 µM) compared with under NES (3.7±2.6 µM) and HES (3.3±2.2 µM). Similar patterns were found in unaffected side that there is significant greater cortical activation of S2 under LES (8.6±1.8 µM) in comparison with those under NES (1.4±1.4 µM) and HES (3.2±1.9 µM).
Figure 1 shows a representative cortical activation patterns in stroke patient with right brain lesion which exhibits greatest cortical activation under LES along with right brain deactivation. General cortical activation is found in NES whereas general deactivation is shown in HES.
DISCUSSION
Previous studies indicate that SMC is involved during cycling exercise [11]. A previous fMRI study also shows that PMC, SMA, SMC, and S2 all participate in wrist movement evoked by neuromuscular electrical stimulation [12]. In our finding, bilateral S2 activation was noticed under all electrical stimulation conditions. However, there is no consisted pattern found in PMC, SMA and SMC comparing the three conditions. This may be resulted from damages of the brain tissue that changes normal cortical activation behavior.
Low-intensity electrical stimulation has been proposed recently to better facilitate muscle activation [13]. It has also been found increase maximal voluntary force of quadriceps in sedentary and poorly performing people through facilitatory neurogenic mechanism [14]. In our investigation, low-intensity of electrical stimulation is found to better facilitate cortical excitability in comparison with high-intensity electrical stimulation, specifically in bilateral S2.
CONCLUSION
Our results show that electrical stimulation of 10 mA could have better facilitation in cortical excitability in comparison with that of 30 mA in stroke patients and no electrical stimulation. Therefore, high-intensity electrical stimulation may not have an improvement on cortical activation. It is interesting that low-intensity rather than high-intensity electrical stimulation is found to have better enhancement on cortical activation in stroke patients.
REFERENCES
[1] J. Lexell and U. B. Flansbjer, "Muscle strength training, gait performance and physiotherapy after stroke," (in eng), Minerva Med, vol. 99, no. 4, pp. 353-68, Aug 2008.
[2] H. Y. Chen, S. C. Chen, J. J. Chen, L. L. Fu, and Y. L. Wang, "Kinesiological and kinematical analysis for stroke subjects with asymmetrical cycling movement patterns," (in eng), J Electromyogr Kinesiol, vol. 15, no. 6, pp. 587-95, Dec 2005.
[3] S. I. Lin, C. C. Lo, P. Y. Lin, and J. J. Chen, "Biomechanical assessments of the effect of visual feedback on cycling for patients with stroke," (in eng), J Electromyogr Kinesiol, vol. 22, no. 4, pp. 582-8, Aug 2012.
[4] P. Y. Lin, J. J. Chen, and S. I. Lin, "The cortical control of cycling exercise in stroke patients: an fNIRS study," (in eng), Hum Brain Mapp, vol. 34, no. 10, pp. 2381-90, Oct 2013.
[5] J. S. Knutson, M. J. Fu, L. R. Sheffler, and J. Chae, "Neuromuscular Electrical Stimulation for Motor Restoration in Hemiplegia," (in eng), Phys Med Rehabil Clin N Am, vol. 26, no. 4, pp. 729-45, Nov 2015.
[6] A. Katz, E. Tirosh, R. Marmur, and J. Mizrahi, "Enhancement of muscle activity by electrical stimulation in cerebral palsy: a case-control study," (in eng), J Child Neurol, vol. 23, no. 3, pp. 259-67, Mar 2008.
[7] P.-W. Hsueh, M.-C. Tsai, and C.-L. Chen, "Stimulation Interval Evaluation for Lower-Limb Cycling Movement Based on Torque Observer," Asian Journal of Control, vol. 20, no. 6, pp. 2318-2330, 2018/11/01 2017.
[8] L. Koessler et al., "Automated cortical projection of EEG sensors: anatomical correlation via the international 10-10 system," (in eng), Neuroimage, vol. 46, no. 1, pp. 64-72, May 15 2009.
[9] T. J. Huppert, S. G. Diamond, M. A. Franceschini, and D. A. Boas, "HomER: a review of time-series analysis methods for near-infrared spectroscopy of the brain," (in eng), Appl Opt, vol. 48, no. 10, pp. D280-98, Apr 1 2009.
[10] D. A. Boas, K. Chen, D. Grebert, and M. A. Franceschini, "Improving the diffuse optical imaging spatial resolution of the cerebral hemodynamic response to brain activation in humans," (in eng), Opt Lett, vol. 29, no. 13, pp. 1506-8, Jul 1 2004.
[11] P. Y. Lin, S. I. Lin, and J. J. Chen, "Functional near infrared spectroscopy study of age-related difference in cortical activation patterns during cycling with speed feedback," (in eng), IEEE Trans Neural Syst Rehabil Eng, vol. 20, no. 1, pp. 78-84, Jan 2012.
[12] A. Blickenstorfer et al., "Cortical and subcortical correlates of functional electrical stimulation of wrist extensor and flexor muscles revealed by fMRI," (in eng), Hum Brain Mapp, vol. 30, no. 3, pp. 963-75, Mar 2009.
[13] E. Langzam, Y. Nemirovsky, E. Isakov, and J. Mizrahi, "Muscle enhancement using closed-loop electrical stimulation: volitional versus induced torque," (in eng), J Electromyogr Kinesiol, vol. 17, no. 3, pp. 275-84, Jun 2007.
[14] P. Valli, L. Boldrini, D. Bianchedi, G. Brizzi, and G. Miserocchi, "Effect of low intensity electrical stimulation on quadriceps muscle voluntary maximal strength," (in eng), J Sports Med Phys Fitness, vol. 42, no. 4, pp. 425-30, Dec 2002.
ACKNOWLEDGEMENTS
This study has been partially supported by grants (107-2221-E-269 -001-MY2 and 107-2221-E-006 -035 -MY3) from Ministry of Science and Technology, Taiwan, ROC.