Qussai M. Obiedat, Maysam M. Ardehali, Brooke C. Follansbee, Roger O. Smith
University of Wisconsin-Milwaukee
ABSTRACT
One of the prevalent disabilities after stroke is the loss of upper extremity motor function, leading survivors to suffer from an increased dependency in their activities of daily living and a general decrease in their overall quality of life. Therefore, the restoration of upper extremity function to improve survivors’ independency is crucial. Conventional stroke rehabilitation interventions, while effective, fall short of helping individuals achieve maximum recovery potential. Functional Electrical Stimulation (FES), both with passive and active approaches, has been found to moderately increase function in the affected limbs. This paper discusses a novel EEG-Based BCI-FES system that provides FES stimulation to the affected limbs based on the brain activity patterns of the patient specifically in the sensory motor cortex, while the patient imagines moving the affected limb. This system allows the synchronization of brain activity with peripheral movements, which may lead to brain reorganization and restoration of motor function by affecting motor learning or re-learning, and therefore induce brain plasticity to restore normal-like brain function.
INTRODUCTION
Stroke is one of the leading causes of severe motor disability, with approximately 800,000 individuals each year are experiencing a new or recurrent stroke in the US alone (1). Advances in healthcare and medical technology, and the high incidence of stroke and its increasing rate in the growing elderly population, have contributed to a relatively large population of stroke survivors currently estimated at 4 million individuals in the United States alone (1). These survivors are left with several devastating long-term neurological impairments.
The most apparent defect after a stroke is motor impairments, with impairment of upper extremity (UE) functions standing as the most disabling motor deficit. Approximately 80% of survivors suffering from UE paresis, and only about one-tenth of the them regain complete functional recovery (2). Stroke survivors generally suffer from a decrease in their quality of life, and an increase dependency in their activities of daily living. Statistically, close to one quarter of the stroke survivors become dependent in activities of daily living (3). Thus, the optimal restoration of arm and hand function is crucial to improve their independence.
Recently, several remarkable advancements in the medical management of stroke have been made. However, a widely applicable or effective medical treatment is still missing, and most post-stroke care will continue to depend on rehabilitation interventions (4). The available UE stroke rehabilitation interventions can be categorized as: conventional physical and occupational therapy, constraint-induced movement therapy (CIT), functional electrical stimulation (FES), and robotic-aided and sensor-based therapy systems (5). Although an increased effort has been made to enhance the recovery process following a stroke, survivors generally do not reach their full recovery potential. Thus, the growing population of stroke survivors is in a vital need for innovative strategies in stroke rehabilitation, especially in the domain of UE motor rehabilitation. This paper presents an innovative integration of a brain-computer interface (BCI) system to actively control the delivery of FES. Early research and product development activities are advancing the reality of this becoming a mainstream intervention option.
PASSIVE VS. ACTIVE DELIVERY OF FES
The use of FES on the impaired arm is an accepted intervention for stroke rehabilitation aiming to improve motor function. A systematic review with meta-analysis of 18 randomized control trials found that FES had a moderate effect on activity compared with no intervention or placebo and a large effect on UE activity compared to control groups, suggesting that FES should be used in stroke rehabilitation to improve the ability to perform activities (6). Specifically, improvements in UE motor function after intensive FES intervention can be ascribed to the increased ability to voluntarily contract impaired muscles, the reduction in spasticity and improved muscle tone in the stimulated muscles, and the increased range of motion in all joints (7). These improvements in UE after FES could be attributable to multiple neural mechanisms, with one mechanism suggesting that proprioceptive sensory input and visual perception of the movement could promote neural reorganization and motor learning (8). A potential limiting factor to the application of FES is that the stimulation is administered manually, usually from a therapist, without any regard to the concurrent brain activity of the patient. This makes the delivery a passive process with no to minimal coordination with the mental task required to happen concurrently from the patient.
On the other hand, electromyography (EMG)-triggered FES systems made the delivery of FES an active process. Such systems are activated through detecting a preset electrical threshold in certain muscles, which provide the user (patient) the ability to actively control the delivery of FES and make the delivery concurrent with the patient’s brain activity. However, a systematic review of 8 randomized controlled trials (n=157) that assessed the effects of EMG-triggered neuromuscular electrical stimulation for improving hand function in stroke patients found no statistically significant differences in effects when compared to patients receiving usual care (9). A possibility to explain the shortcoming of EMG-triggered FES systems, is that the ability of the brain to generate and send efficient neural signals to the peripheral nervous system is disrupted after stroke, which could affect the control mechanism of these systems. Thus, the synchronization of FES with brain activity maybe critical for the optimization of recovery.
AN ACTIVE EEG-BASED BCI-FES SYSTEM
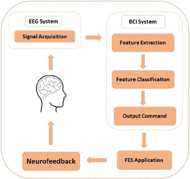
BCI technology can be used to actively control the FES application through detecting the brain neural activity directly when imagining or attempting a movement. Performing or mentally imagining (or as it commonly called motor imagery) a movement results in the generation of neurophysiological phenomena called event-related desynchronization or synchronization (ERD or ERS). ERD or ERS can be observed from Mu (9–13 Hz) or Beta rhythms (22–29 Hz) over the primary sensorimotor area contralateral to the imagined part of the body (10). These rhythms can be detected using electroencephalography (EEG). Therefore, an EEG based BCI system can be utilized to provide automated FES neurofeedback through detecting either actual movement or motor imagery (MI) and can be used to train the voluntary modulation of these rhythms. The ability to modulate these rhythms alongside the real-time neurofeedback from the FES application may induce neuroplastic change in a disrupted motor system to allow for more normal motor-related brain activity, and thus promote functional recovery. Figure 1 provides an overview of the BCI-FES system.
Any BCI-FES intervention session includes two screening tasks: an open-loop screening followed by a closed-loop task. The open-loop screening task is used to identify appropriate EEG-based control features to guide all subsequent closed-loop tasks. In the open-loop screening task, subjects are instructed to perform attempted movement of either hand by following on-screen cues of “right”, “left”, and “rest”. The attempted movement can vary across subjects, depending on the subject’s baseline abilities and recovery goals. For example, subjects can perform opening and closing of the hand or wrist flexion/extension movements. During this screening task, no feedback is provided to the subject.
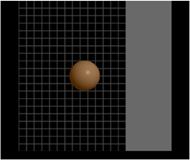
Data from the open-loop screening task will then be analyzed to identify appropriate EEG-based control features by determine the EEG channels the presents the largest r-squared values within the frequency ranges of the Mu and Beta rhythms for each attempted movement using left or right hand (11). The identified channels and the specific frequency bins will then be used to control the signals for the closed-loop neurofeedback task.
In the closed-loop screening task, a real-time visual feedback is given to the subject in a form of a game. A ball appears on the center of a computer monitor with a vertical rectangle (target) to either the right or left side of the screen (Figure 2). The movement of the ball is controlled by the BCI system in which the detection of an attempted movement in either hand will be translated into moving the ball toward the same side. For example, if the target appeared on the left side of the screen and the BCI system detected a movement attempt of the user’s left hand, the ball then moves toward the left. Users are instructed to perform or attempt the same movement that they used during the open-loop task. The FES electrodes are placed on the subject’s affected side over a specific muscle of the forearm. The selection of which muscle to be innervated with FES is dependent on the rehabilitation goal for the subject. For example, if a subject is having a difficulty extending his/her wrist, the FES electrodes are placed over the extensor muscles of the impaired forearm.
The FES neurofeedback is triggered when cortical activity related to attempted movement of the impaired limb is detected by the BCI system, and the subject is cued to attempt movement of the impaired hand. Thus, since both ball movement and FES are controlled by the same set of EEG signals, FES is only applied when the ball moves correctly toward the target on the affected side of the body. This triggering of the FES ensures that only consistent, desired patterns of brain activity associated with attempted movement of the impaired hand are rewarded with feedback from the FES device.
DISCUSSION
The growing population of stroke survivors constitutes an increasing need for new strategies in stroke rehabilitation. Thus, it is imperative to explore novel intervention technologies that present promise to aid in the recovery process of this population. Some studies suggest that noninvasive EEG-based BCI systems hold a potential for facilitating upper extremities motor recovery after stroke (12,13). Although several groups had gave up on the idea of using non-invasive EEG-based BCI systems for control, there might be several implementations of these systems in the context of rehabilitation that yet need to be explored. The active EEG-based BCI-FES system is one example. However, more research and clinical studies are needed to investigate the efficacy of the system in order to be accepted and integrated into regular stroke rehabilitation practice.
REFERENCES
(1) Norrving B, Kissela B. The global burden of stroke and need for a continuum of care. Neurology 2013 Jan 15;80(3 Suppl 2):S5-12.
(2) Langhorne P, Coupar F, Pollock A. Motor recovery after stroke: a systematic review. The Lancet Neurology 2009;8(8):741-754.
(3) Sanchez RJ, Liu J, Rao S, Shah P, Smith R, Rahman T, et al. Automating arm movement training following severe stroke: functional exercises with quantitative feedback in a gravity-reduced environment. IEEE Transactions on neural systems and rehabilitation engineering 2006;14(3):378-389.
(4) Langhorne P, Bernhardt J, Kwakkel G. Stroke rehabilitation. The Lancet 2011;377(9778):1693-1702.
(5) Loureiro RC, Harwin WS, Nagai K, Johnson M. Advances in upper limb stroke rehabilitation: a technology push. Med Biol Eng Comput 2011;49(10):1103.
(6) Howlett OA, Lannin NA, Ada L, McKinstry C. Functional electrical stimulation improves activity after stroke: a systematic review with meta-analysis. Arch Phys Med Rehabil 2015;96(5):934-943.
(7) Kawashima N, Popovic MR, Zivanovic V. Effect of intensive functional electrical stimulation therapy on upper-limb motor recovery after stroke: case study of a patient with chronic stroke. Physiotherapy Canada 2013;65(1):20-28.
(8) Wang R. Neuromodulation of effects of upper limb motor function and shoulder range of motion by functional electric stimulation (FES). Operative Neuromodulation: Springer; 2007. p. 381-385.
(9) Meilink A, Hemmen B, Seelen H, Kwakkel G. Impact of EMG-triggered neuromuscular stimulation of the wrist and finger extensors of the paretic hand after stroke: a systematic review of the literature. Clin Rehabil 2008;22(4):291-305.
(10) Ang KK, Guan C. EEG-Based Strategies to Detect Motor Imagery for Control and Rehabilitation. IEEE Transactions on Neural Systems and Rehabilitation Engineering 2017;25(4):392-401.
(11) Wilson JA, Schalk G, Walton LM, Williams JC. Using an EEG-based brain-computer interface for virtual cursor movement with BCI2000. J Vis Exp 2009 Jul 29;(29). pii: 1319. doi(29):10.3791/1319.
(12) Caria A, Weber C, Brötz D, Ramos A, Ticini LF, Gharabaghi A, et al. Chronic stroke recovery after combined BCI training and physiotherapy: a case report. Psychophysiology 2011;48(4):578-582.
(13) Young BM, Nigogosyan Z, Remsik A, Walton LM, Song J, Nair VA, et al. Changes in functional connectivity correlate with behavioral gains in stroke patients after therapy using a brain-computer interface device. Frontiers in neuroengineering 2014;7:25.
ACKNOWLEDGMENT
This project is supported in part by UW-Madison Institute for Clinical and Translational Research, and College of Health Sciences, UW-Milwaukee.