Hala E.Osman1, Debbie Espy2, Antonie van den Bogert3, Ann Reinthal2
1Cleveland State University, Department of Biomedical Engineering, Cleveland, OH, USA, 2Cleveland State University, School of Health Sciences, Cleveland, OH, USA,3Cleveland State University, Department of Mechanical Engineering, Cleveland, OH, USA
INTRODUCTION
Falls are the most common and costly medical complication in the stroke population[1,2] and fear of falling is one of the problems that stroke survivors encounter on a daily basis. The high fall risk for people who have had a stroke has not only mental but significant physical consequences such hip fractures and limited mobility.[3] In neurological pathologies such as stroke, hemiparesis (i.e., weakness of one side of the body), sensory impairments, and cognitive impairments are major effects [4], all of which negatively impact balance control. Maintaining balance is one of the major challenges for human movement control and, particularly among those who have had a stroke, it is essential to restore stability (i.e. the ability to maintain balance to prevent falling).[5]
Reactive balance can be defined as a response to unexpected perturbation to stability whereas proactive balance is anticipatory responses based on what the specific tasks require.[6] Reactive balance control is important due to the role it plays in the voluntary movements that are fundamental to stabilizing the body when perturbations occur. It has been suggested that balance training should involve reactive responses to sudden external gait perturbations, considered to be task- specific and more effective than general exercise interventions for fall prevention.[7] Furthermore, it has been established that external perturbations have an effect on both body position and velocity of the center of mass (COM) relative to foot stance.
During a dynamic activity like walking, the body is in a continuous imbalance because the COM does not stay within the base of support (BOS) of the feet. To recover from an unexpected displacement of the COM, movements that bring the COM back within the stability limits determined by the BOS are required. This is further complicated among people who have had a stroke because of differences between the paretic and non-paretic limbs during walking.[4] Reactive steps are required to prevent falls when gait is perturbed. However stroke participants have difficulty initiating and executing successful steps.[8] It has been suggested that impaired stepping in reactive balance among people who have had a stroke is strongly correlated with their risk of falls. Fall risk assessment for people post stroke is clinically essential given that falls for this group has an incidence of 50 - 70%.[9] Understanding the mechanisms underlying recovery from a stroke is clinically significant as it serves as the foundation for developing functional rehabilitation strategies that can offer stroke survivors a greater possibility of improvement.[10]
Treadmill perturbation-based balance assessment can make unique contributions to fall prevention and balance control strategies as it incorporates techniques of physical therapy examination and biomechanical assessment. Despite the large body of studies that focus on gait stability, the ways to quantify the dynamic stability of human gait is still not fully clear. Thus, there is a need to develop such stability measures, particularly for the post stroke population. Therefore, the main goal of this paper was to develop gait perturbation- based biomechanical assessments for people who have had a stroke. It hypothesized that using treadmill-based perturbation balance assessment will allow both engineers as well as physical therapist to accurately assess fall prevention and balance control strategies.
METHODS
A randomized treadmill gait perturbation-based balance assessment on the paretic limb was developed and used for pre/post-tests. Each trial consisted of three periods: normal walking speed, perturbation induction, and recovery (max. of 15 trials). At the end of each trial participants were asked to rate their perceived stability using a scale that was developed by our lab.[11]
Motion capture system
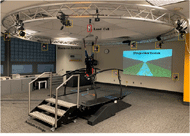
1) R- Mill treadmill (Forcelink, Culemborg, Netherlands) with 6 degree of freedom force plates, independent belt for each foot.
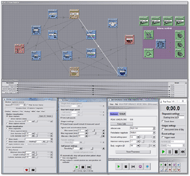
In addition, D-flow is responsible for controlling the treadmill speed and motion. We have created a computer code using scripting language Lua to control the treadmill when running the whole study protocol as illustrated in Figure 2.
3) Cortex software (Motion Analysis. Santa Rosa, CA, USA) is used to deliver the 3D marker trajectories from the 10 cameras and the force plates as well as data from the load cell that reflect bodyweight.
4) 10 Osprey cameras (Motion Analysis. Santa Rosa, CA, USA) motion capture system paired with cortex software. The coordinate system in the direction of walking is (x-right, y -upwards, and z-backwards). The point that located in the center of the treadmill surface serves as the origin for both the camera and ground force reaction coordinate systems that used to calibrate the system prior testing.
Finding walking speed
The subjects were asked to simply walk at their comfortable, natural, but safe speed to find the normal walking speed in m/s. Then subjects were asked to walk as fast as they can to find their maximum walking speed in m/s.
After the walking tasks, forty-seven reflective markers were placed on anatomical landmarks on the body according the full-body marker model.
The subject is guided off the treadmill to rest while marker reconstruction takes place. Once the markers have been reconstructed, the subject is led back on the treadmill, and secured to the safety bar.
Perturbation Magnitudes (mPer)
Perturbation occurred once per trial, at the mid- stance of the hemi-paretic lower extremity. The initial perturbation magnitude was based on the score of Mini Balance Evaluation System test (MiniBEST). Participants walked at their normal walking speed during testing. The intensity of the external perturbation was calculated as the percentage of participant’s maximum walking speed. Therefore, perturbation magnitudes were categorized as small (55%), Medium (75%), and High (95%). To ensure safety, participants wore a safety harness attached to the swivel rod end centered above the treadmill.
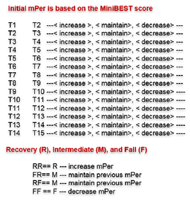
To eliminate the human factor error and to ensure reliability of the outcome measures, a tension / compression load cell (LCCD-1K), connected to the harness, was used to quantify the maximum bodyweight (BW) during the perturbation to determine whether it was a recovery (> 30% of BW), fall (< 5% of BW) or intermediate.[12,13] To calibrate the load cell, known weights were used as an input to calculate the voltage value, the gain then was calculated as the known weight divided by the voltage. After the installation of the load cell, an analog channel was added into the data acquisition (DAQ) to display the percentage of the peak load during testing.
RESULTS
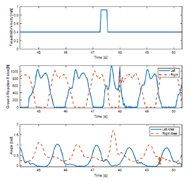
LIMITATIONS
One of the study limitations is that it was not feasible to assess post stroke individuals to find their normal and maximum speed by using the self-paced function, in which participant determines their own walking speed in the treadmill. That due to the difference in gait characteristics between the paretic and non- paretic sides during walking.[4]
Another limitation might be due to treadmill system sensitivity: since the perturbation magnitude is directly proportional to maximum walking speed, when the maximum speed is very small, the perturbation may not be detected.
CONCLUSIONS
The main objective of this method paper was to describe our developed treadmill gait perturbation-based biomechanical assessment for post stroke survivors. This work seeks to open new avenues for exercise interventions for the stroke population. Treadmill gait perturbation provides unexcepted perturbations to avoid predictability, yet offer highly repeatable and reproducible measures and also to give a complete body motion to mimic an actual trip.[14,15] Thus, biomechanical assessments offer a promising approach not only in developing prevention strategies but in identifying people who are at risk of falling. The information obtained from this work may contribute to reducing the risk of falls in the post stroke population and improve their overall health.REFERENCES
[1] Honeycutt CF, Nevisipour M, Grabiner MD. Characteristics and adaptive strategies linked with falls in stroke survivors from analysis of laboratory-induced falls. J Biomech. 2016;49(14):3313–9.
[2] Roos PE, Dingwell JB. Using dynamic walking models to identify factors that contribute to increased risk of falling in older adults. Hum Mov Sci. 2013;32(5):984–96.
[3] Weerdesteyn PhD, PT V, de Niet MSc M, van Duijnhoven MSc HJR, Geurts MD, PhD ACH. Falls in individuals with stroke. J Rehabil Res Dev [Internet]. 2008;45(8):1195–213.
[4] Haarman JAM, Vlutters M, Olde Keizer RACM, Van Asseldonk EHF, Buurke JH, Reenalda J, et al. Paretic versus non-paretic stepping responses following pelvis perturbations in walking chronic-stage stroke survivors. J Neuroeng Rehabil. 2017;14(1).
[5] Liu C, Macedo L De, Finley JM. Conservation of Reactive Stabilization Strategies in the Presence of Step Length Asymmetries During Walking. Front Hum Neurosci [Internet]. 2018;12.
[6] Shumway-Cook A, Woollacott MH. Motor control: Translating research into clinical practice: Fourth edition. Motor Control: Translating Research into Clinical Practice: Fourth Edition. 2014. 1-641 p.
[7] McCrum C, Gerards MHG, Karamanidis K, Zijlstra W, Meijer K. A systematic review of gait perturbation paradigms for improving reactive stepping responses and falls risk among healthy older adults. Vol. 14, European Review of Aging and Physical Activity. 2017.
[8] Mansfield A, Wong JS, McIlroy WE, Biasin L, Brunton K, Bayley M, et al. Do measures of reactive balance control predict falls in people with stroke returning to the community? Physiother (United Kingdom). 2015;101(4):373–80.
[9] Lee HH, Jung SH. Prediction of Post-stroke Falls by Quantitative Assessment of Balance. Ann Rehabil Med [Internet]. 2017;41(3):339–46.
[10] Geurts ACH, De Haart M, Van Nes IJW, Duysens J. A review of standing balance recovery from stroke. Vol. 22, Gait and Posture. 2005. p. 267–81.
[11] Espy D, Reinthal A, Meisel S. Intensity of Balance Task Intensity, as Measured by the Rate of Perceived Stability, is Independent of Physical Exertion as Measured by Heart Rate. Vol. 7, Journal of Novel Physiotherapies. 2017.
[12] Yang F, Bhatt T, Pai YC. Role of stability and limb support in recovery against a fall following a novel slip induced in different daily activities. J Biomech. 2009;42(12):1903–8.
[13] Bhatt T, Espy D, Yang F, Pai YC. Dynamic gait stability, clinical correlates, and prognosis of falls among community-dwelling older adults. Arch Phys Med Rehabil. 2011;92(5):799–805.
[14] Yang F, Bhatt T, Pai YC. Generalization of treadmill-slip training to prevent a fall following a sudden (novel) slip in over-ground walking. J Biomech. 2013;46(1):63–9.
[15] Sessoms PH, Wyatt M, Grabiner M, Collins JD, Kingsbury T, Thesing N, et al. Method for evoking a trip-like response using a treadmill-based perturbation during locomotion. J Biomech. 2014;47(1):277–80.