J. Rippetoe1,2
, S. Brown
1,2
, M. Shuping
1,2
, M. Foote
1,2
, L. Wilson
1,3
, C. Dionne
1,3
, H. Wang
1,2
1
University of Oklahoma Health Sciences Center,
2
Technology for Occupational Performance Laboratory,
3
Center for Human Performance Measurement
INTRODUCTION
Approximately 70% of individuals with diabetes develop diabetic peripheral neuropathy (DPN). [1] The high costs related to DPN and its complications in the U.S. were estimated to be between $4.6 and $13.7 billion. [2] DPN impacts overall function and causes other health risks, e.g., enhanced risk for injurious falls. [3] Studies have shown that DPN causes loss of cutaneous sensation and proprioception in the legs, toes, arms, and hands, which leads to alterations in gait, measured by spatiotemporal, kinematic, and kinetic parameters. [3-5] When compared to otherwise healthy adults, individuals with DPN demonstrate slower gait speed, decreased step length, increased stance period, increased duration in double support, and increased step time. [4] Further, individuals with DPN demonstrate a relative decreased kinematic and kinetic measures, specifically in maximum dorsiflexion, ankle sagittal range of motion, maximum knee flexion, and peak plantar flexor moment. [4-5] The changes in these gait parameters have been linked to an increased number of fall events and risk of injurious falls. [4-5]
Multiple approaches have been proposed to treat or manage the gait impairments of DPN, e.g., whole-body vibration (WBV), transcutaneous electrical nerve stimulation, etc., yet there is no consensus among their respective outcomes. [6-7] Mechanical stimulation in the form of whole-body vibration (WBV) has gained popularity in the fields of exercise physiology, sports performance, and rehabilitation. [8] While WBV has potential benefit in patients with DPN including improving gait for individuals with DPN, drawbacks like tissue inflammation and potential for adverse side effects on the neurological and vascular systems have not been resolved. [6] Focal vibration (FV) therapy has been a useful tool in neurorehabilitation by greatly improving gait performance for patients with other conditions, such as stoke and spinal cord injuries. [9] Further, unlike WBV, focal vibration applied to specific muscles or tendons might be kept within safe and comfortable limits.
Therefore, the purpose of this study was to investigate FV as an intervention to improve gait performance in individuals with DPN. We hypothesized that the gait parameters affected by DPN would improve after FV therapy.
METHODS
Participants
We piloted a single group pre-post, 4-week, at-home study. Ambulatory participants with type 2 diabetes with DPN were recruited for the study. An "Individual with DPN" was defined as having an insensitivity to a 10-gram monofilament sensation testing at 1-3 sites in any of the following locations in either foot: hallux, 1st, 3rd, or 5th metatarsal heads. The inclusion criteria were: 1) diagnosis of diabetes for at least one year; 2) diabetic peripheral neuropathy as defined by failure to sense the 5.07 (10g) monofilament test in one or more of the six sites tested; 3) age 18-75 years old; 4) able to ambulate independently without assistive devices (e.g. walker or crutches) for 30 feet; 5) no evidence of neurological (other than peripheral neuropathy) or orthopedic conditions; 6) able to understand English instructions; and 7) have normal or corrected vision. Individuals with other non-diabetic causes of neuropathy by history, symptomatic peripheral vascular disease, joint pain, swelling and/or limited of range of motion in the lower extremities that interfere with walking, other systemic or local diseases that could interfere with walking assessment, amputation in the lower extremities, clinically diagnosed with dementia greater than mild (screened using Montreal Cognitive Assessment (MOCA) <24) were excluded. A physician or therapist with relevant training in DPN care confirmed eligibility of subjects. All subjects interested in the study were informed about the procedure and the aim of the research. Eligible and willing patients signed an institutional review board approved informed consent prior to screening. We utilized Timed-Up-and-Go (TUG) and TUG Cognitive tests to further investigate whether individuals with DPN that were at a fall risk could further benefit from the FV therapy.
Protocol
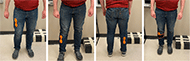
After obtaining consent and screening approval, participants wore wearable FV devices to the three muscles most relevant for gait, i.e., quadricep, gastrocnemius, and tibialis anterior, on each leg for 10 minutes per muscle daily for 3 days/week over 4 weeks (Figure 1). Participants were provided instructions on how to utilize and apply the device. TUG and TUG cognitive tests were conducted at baseline to determine whether any individuals were at a fall risk. Spatial-temporal, kinematic, and kinetic data were collected at baseline and the end of the 4-week at the Center for Human Performance Measurement with Qualysis™ motion capture system and AMTI™ force plates. Each participant underwent 5 trials at a self-selected speed at each visit. Demographic data on age, gender etc. were collected at the first visit.
Data analysis
Spatial-temporal, kinematic, and kinetic parameters that were impaired due to DPN were the only gait parameters that were analyzed. Data were analyzed using Visual3D© and Excel™. Descriptive statistics and paired t-tests were used for data analysis.
RESULTS
13 participants have completed the study to date. TUG and TUG Cognitive scores indicate that seven participants (54%) are at a fall risk (TUG score>13.5 seconds and TUG Cognitive score>15 seconds) (Table 1). [10] The data from each of the five trials were averaged for each participant and then were averaged over all the participants. It was noted that one participant failed to comply with the at-home treatment regimen by missing treatment sessions due to cognitive and memory problem. Another participant had an accident in the middle of the study that significantly impacted their walkability. Therefore, data without those two participants were analyzed, which showed a significant improvement in right step length, left and right step time, and left knee maximum flexion (p<0.05) and trending toward significant improvement in right knee maximum flexion (p=0.061) after the 4-week treatment (Table 2). Patients who were at a fall risk (n=5, excluding pts. 6 and 14) were further analyzed separately and showed significant improvement in gait speed, left step time, left stance time, double limb support, right maximum plantarflexion, and right peak plantar flexor moment (p<0.05), and trending toward significant improvement in right step time (p=0.075) after the 4-week treatment (Table 2).
Pt ID | Age (years) | Gender | Weight (lbs) | Height (inches) | Ethnicity | No. of years with Diabetes | TUG (s) | TUG Cognitive (s) |
---|---|---|---|---|---|---|---|---|
1 | 63 | Male | 245 | 69 | Caucasian | 10 | 5.5 | 7 |
2 | 72 | Female | 235 | 68 | Caucasian | 11 | 13.47 | 14.36 |
3 | 74 | Female | 258 | 68 | Caucasian | 21 | 15.5* | 15.5* |
4 | 79 | Male | 178 | 70 | Caucasian | 5 | 10.5 | 12 |
5 | 53 | Female | 343 | 66 | African-American | 23 | 16.5* | 21* |
6 | 70 | Female | 130 | 66 | Caucasian | 19 | 13.93* | 13.69 |
7 | 55 | Female | 197 | 64 | Caucasian | 22 | 12.35 | 14.87 |
8 | 70 | Female | 210 | 64 | Caucasian | 19 | 12.5 | 16.81* |
9 | 67 | Male | 355 | 72 | Caucasian | 2 | 13.68* | 11.05 |
10 | 74 | Female | 166 | 60.5 | Caucasian | 11 | 22.06* | 26.12* |
11 | 55 | Female | 212 | 65 | Eurasian | 15 | 9.82 | 12.09 |
12 | 63 | male | 217 | 71 | Caucasian | 7 | 8.34 | 10.38 |
13 | 59 | Female | 120 | 62 | Caucasian | 19 | 18.38* | 20.78* |
Gait Parameter | 11 Participants | Fall Risk Group | ||
---|---|---|---|---|
Baseline | 4-weeks | Baseline | 4-weeks | |
Gait speed (m/s) | 0.947 (0.387) | 1.005 (0.215) | 0.749 (0.149) | 0.829 (0.116)* |
Left step length (m) | 0.519 (0.095) | 0.544 (0.079) | 0.450 (0.058) | 0.480 (0.025) |
Right step length (m) | 0.521 (0.106) | 0.561 (0.109)* | 0.455 (0.058) | 0.474 (0.062) |
Left step time (s) | 0.600 (0.047) | 0.555 (0.044)* | 0.596 (0.048) | 0.571 (0.046)* |
Right step time (s) | 0.612 (0.064) | 0.564 (0.049)* | 0.637 (0.072) | 0.594 (0.047)** |
Left stance time (s) | 0.765 (0.130) | 0.728 (0.073) | 0.843 (0.101) | 0.772 (0.077)* |
Right stance time (s) | 0.732 (0.145) | 0.705 (0.068) | 0.753 (0.131) | 0.722 (0.085) |
Double support duration (s) | 0.368 (0.081) | 0.332 (0.051) | 0.414 (0.067) | 0.361 (0.055)* |
Left Maximum dorsiflexion (degrees) | 20.696 (2.725) | 19.986 (2.943) | 21.950 (2.275) | 21.253 (3.650) |
Right Maximum dorsiflexion (degrees) | 20.840 (1.751) | 20.278 (2.077) | 21.794 (1.819) | 21.524 (2.267) |
Left Maximum plantarflexion (degrees) | 13/986 (5.989) | 14.831 (6.779) | 9.724 (3.135) | 12.485 (9.025) |
Right Maximum plantarflexion (degrees) | 12.723 (8.658) | 12.373 (5.695) | 6.464 (5.669) | 8.388 (5.432)* |
Left ankle ROM (degrees) | 34.682 (6.023) | 34.817 (5.091) | 31.673 (3.467) | 33.737 (6.377) |
Right ankle ROM (degrees) | 33.563 (8.826) | 32.651 (5.514) | 28.257 (6.687) | 29.912 (5.954) |
Left maximum knee flexion (degrees) | 54.072 (6.794) | 60.200 (7.612)* | 50.849 (4.331) | 56.474 (6.105) |
Right maximum knee flexion (degrees) | 49.463 (13.525) | 55.403 (13.576)** | 52.047 (6.053) | 57.997 (8.557) |
Left peak plantar flexor moment (N*m/Kg) | 0.976 (0.403) | 1.019 (0.383) | 0.742 (0.441) | 0.796 (0.429) |
Right peak plantar flexor moment (N*m/Kg) | 1.032 (0.281) | 1.038 (0.206) | 0.822 (0.217) | 0.909 (0.222)* |
DISCUSSION
This is the first study to our knowledge to investigate the effects of FV on gait impairments in DPN. Therefore, we could not compare our results to any published data. This study evaluated the effect of FV therapy on spatial-temporal, kinematic, and kinetic gait parameters in patients with DPN. The emerging results of this study appears to partially confirm our ongoing hypothesis: FV therapy would improve spatial-temporal, kinematic, and kinetic gait parameters that were impaired by DPN. When looking at the data without the two patients having trouble to follow the study instructions, significantly improvement on left knee flexion and marginally right knee flexion were noted, which were closer to healthy control knee flexion range. [4-5] These findings indicate that FV therapy improves knee range of motion but due to the small sample size other spatial-temporal, kinematic, and kinetic gait data were again, not improved to allow us to determine the mechanism of how FV improves gait in DPN. However, a possibility for why the kinetic and kinematic data were not improved may be that FV therapy slowed the progression of the patients' DPN and thus, may have postponed the worsening of the patients' gait performance. Further, FV increased right plantarflexion and the peak plantar flexor moment for the fall risk group, which indicates that FV increased the muscular strength of the plantar flexor muscles, which may have allowed for greater push-off with the right foot and thus, improved gait speed.
There were several limitations to our study. First, a control group has not be included in the ongoing study at this time. While we will include control group and other intervention groups in the future studies, the preliminary results from this study will contribute to the calculation of effect sizes. Second, this study had a small sample size but this is an ongoing study and our targeted sample size is 30. Third, although participants were instructed on where the device should be applied, the placement of the device on the tibialis anterior, i.e., the muscle for dorsiflexion, may not have been adequately placed due to the difficulty of placing a device on a smaller muscle. Future design improvement will be needed to make it easier for the participant to wear the device. Last, since the intervention was completed at home, we were unable to ensure that participants complied with the established protocol. However, we are working with our industrial partners on an updated version of the technology which will enable us to track the vibration usage through an app. Despite the study limitations and need for more recruitment and data analysis, our preliminary data suggests that FV therapy may be a useful treatment for DPN-related gait impairment. Our results also highlight the importance in the development of FV devices that can record the usage of the FV therapy for individuals with DPN.
CONCLUSION
Despite only the fall risk group acquiring significant improvement in kinematics and kinetics of gait performance, participants with DPN demonstrated significant improvements in several spatial-temporal measures of gait after 4 weeks of using FV. More data with larger samples will be needed to further confirm and understand the gait improvements.
We are grateful to the participants who gave their time and to the Department of Rehabilitation Sciences, Technology for Occupational Performance Laboratory for the use of their facilities. This work supported in part by an Exploratory Grant award from Harold Hamm Diabetes Center at the University of Oklahoma.
REFERENCES
- Haslbeck, M. (2010). Diabetic neuropathies (part 2): complications, comorbidities and etiology. MMW Fortschritte der Medizin, 152(11), 45-48.
- Gordois, A., Scuffham, P., Shearer, A., Oglesby, A., & Tobian, J. A. (2003). The health care costs of diabetic peripheral neuropathy in the U.S. Diabetes Care, 26(6), 1790–1795. https://doi.org/10.2337/diacare.26.6.1790
- Harati, Y. (2010). Diabetic Peripheral Neuropathies. Methodist DeBakey Cardiovascular Journal, 6(2), 15–19. https://doi.org/10.14797/mdcj-6-2-15
- Fernando, M., Crowther, R., Lazzarini, P., Sangla, K., Cunningham, M., Buttner, P., & Golledge, J. (2013). Biomechanical characteristics of peripheral diabetic neuropathy: A systematic review and meta-analysis of findings from the gait cycle, muscle activity and dynamic barefoot plantar pressure. Clinical Biomechanics, Vol. 28, pp. 831–845. https://doi.org/10.1016/j.clinbiomech.2013.08.004
- Hazari, A., Maiya, A. G., Shivashankara, K. N., Agouris, I., Monteiro, A., Jadhav, R., … Mayya, S. S. (2016). Kinetics and kinematics of diabetic foot in type 2 diabetes mellitus with and without peripheral neuropathy: a systematic review and meta-analysis. SpringerPlus, Vol. 5, pp. 1–19. https://doi.org/10.1186/s40064-016-3405-9
- Hong, J., Barnes, M. J., & Kessler, N. J. (2015). Case study: Use of vibration therapy in the treatment of diabetic peripheral small fiber neuropathy. International Journal of Diabetes Mellitus, 3(1), 72–75. https://doi.org/10.1016/j.ijdm.2011.01.010
- Antony T, & Merghani TH. (2015). The Effectiveness of Transcutaneous Electric Nerve Stimulation (TENS) in The Management of Painful Diabetic Peripheral Neuropathy (DPN)-A critiquing. IOSR Journal of Dental and Medical Sciences (IOSR-JDMS) e-ISSN, 14(10), 85–90. https://doi.org/10.9790/0853-1410108590
- Rittweger, J. (2010, March 12). Vibration as an exercise modality: How it may work, and what its potential might be. European Journal of Applied Physiology, Vol. 108, pp. 877–904. https://doi.org/10.1007/s00421-009-1303-3
- Murillo, N., Valls-Sole, J., Vidal, J., Opisso, E., Medina, J., & Kumru, H. (2014). Focal vibration in neurorehabilitation. European Journal of Physical and Rehabilitation Medicine, Vol. 50, pp. 231–242. Edizioni Minerva Medica.
- Shumway-Cook, A., Brauer, S., & Woollacott, M. (2000). Predicting the Probability for Falls in Community-Dwelling Older Adults Using the Timed Up & Go Test. Physical Therapy, 80(9), 896–903. https://doi.org/10.1093/ptj/80.9.896