R. Goettl1
, T. Ammon
1
, C. Brown
1
1
Midwestern University - Glendale
INTRODUCTION
Strokes are the leading cause of long-term disability in the United States. The most common impairment after stroke is hemiparesis of the contralateral upper limb, with more than 80% of stroke patients experiencing this condition acutely and more than 40% chronically [1]. Residual upper extremity impairments that induce disability in functional performance of ADLs remain in up to 66% of individuals [2]. In fact, only 5 to 20% of patients demonstrate a complete functional recovery of the upper limb [3]. As a result of the prevalence in residual upper extremity motor impairment, along with the several limitations of conventional rehabilitation treatment methods, novel strategies targeting upper limb motor recovery are needed. One such emerging strategy is virtual reality-based intervention.
Virtual Reality (VR) based treatment is a computer-based technology that promotes use of the body to interact with a simulated multisensory environment [4]. The efficacy of VR based interventions is already well researched and supported within the literature [4,5,6]. However, it remains less clear whether the type of VR system that is utilized influences the extent of recovery [8]. Presently, the available literature divides these systems into two categories: Specific and nonspecific. Specific VR systems are specifically designed to be used for rehabilitation, while nonspecific systems include commercial or consumer-based products, like gaming consoles. Due to the beforementioned concerns regarding conventional stroke rehabilitation methods and the potential implications of VR based interventions, this review and meta-analysis sought to determine the difference in efficacy between specific and nonspecific VR systems in order to maximize post stroke functional recovery.
METHODS
Inclusion/exclusion criteria
The following inclusion criteria were established to be utilized for this review:
- Article design must be a systematic review or meta-analysis. This criterion was established as a result of the abundance of available research, in order to only consider the highest level of evidence.
- Interventions in studies included by review must target upper extremity rehabilitation, post stroke and classify virtual reality-based system as specific or non-specific
- Include data of individual studies that could be used in a meta-analysis
- Article must be published in English
Articles that compared virtual reality-based interventions to alternative treatments other than conventional therapy, or in addition to alternative treatments other than conventional therapy, were excluded from this review.
Search strategy and Selection Method
A search of the literature was performed in September 2020 using the following databases: Pubmed, CINAHL, and ERIC. Searches were conducted using the following combination of keywords/MeSH descriptors: "Stroke AND Virtual Reality AND Upper Extremity". The reference lists and related studies of articles identified from the search results were utilized in finding additional potential articles.
Potentially relevant articles were first identified and selected based on title. Potential articles were further condensed after reviewing the abstract. Finally, the full texts of remaining articles were reviewed for compliance according to established inclusion and exclusion criteria.
Data abstraction
Two reviewers (T.A and R.G) participated in the study selection process. Each reviewer independently completed data extraction from each study using consistent forms composed of established criteria. Extracted data was then compared between reviewers until a consensus was reached. The quality of each study was evaluated utilizing the Assessment of Multiple Systematic Reviews (AMSTAR). This instrument consists of 11 items designed to measure the methodological quality of systematic reviews with good face and content validity [7].
Data analysis
In order to evaluate the efficacy of specific versus nonspecific VR based interventions and their relative potential implications regarding upper limb stroke motor rehabilitation, a meta-analysis was conducted comparing the effect sizes reported by other meta-analyses. In this analysis, the standardized difference in means was calculated with a 95% confidence interval. The results are displayed in forest plots. All statistical analyses were conducted utilizing the statistical software Comprehensive Meta-Analysis.
RESULTS
Description of the sample
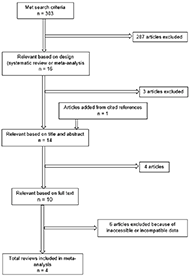
A search of the literature was performed in September 2020. The process is illustrated in Figure 1. Following the selection process, a total of 4 studies contributed to our meta-analysis for statistical comparison.
Overview of the findings
Among the 4 systematic reviews included in this meta-analysis, there were a total of 66 individual studies. The characteristics of these 4 reviews can be found in Table 1.
Maier and colleagues [9] contributed data from 30 individual studies. 2 individual studies were excluded because comparable data was not reported or available. Laver and colleagues [10] contributed data from 14 individual studies. 9 individual studies were excluded as they were duplicates and had already been contributed from previous studies. Lee and colleagues [11] contributed data from 18 individual studies. 3 individual studies were excluded as duplicates. Dominguez-Tellez and colleagues [12] contributed data from 4 individual studies. 8 individual studies were excluded as duplicates and 1 study was excluded because it was not reported whether they utilized Specific or Nonspecific VR interventions and could not be grouped accordingly.
Author & Year | # of studies | Dates of Analysis | VR Systems Used (Specific vs Nonspecific) | Inclusion Criteria | Chronic vs Acute | Fugl Meyer | Wolf Motor Function Test |
---|---|---|---|---|---|---|---|
Maier et al., 2019 | 30 | Inception until August 2018 | Specific and Nonspecific | RCTs that tested the efficacy of SVR or NSVR systems Recovery of the upper limb Patients in the acute, subacute, or chronic | Acute, Subacute, and Chronic included but not analyzed separately | Yes | Yes |
Laver et al., 2017 | 50 | 2004 to 2016 | Nonspecific only | 18 years and older All types of strokes, all levels of severity, and at all stages post stroke | Subacute vs Chronic analyzed | Yes | Yes |
Lee et al., 2019 | 21 | January 2000 to June 2018 | Specific and Nonspecific | Patients diagnosed with chronic stroke Studies using VR as a therapeutic intervention Studies that measured function-related changes RCTs | Chronic only | Yes | Yes |
Domínguez-Téllez et al., 2020 | 15 | 2007 to 2018 | Specific and Nonspecific | Articles published in the last 10 years (2007-2018) English or Spanish language RCTs Adult patients with stroke Outcomes related to motor function of UL and quality of life | Acute and Chronic Included Not analyzed separately | Yes | No |
Data analysis
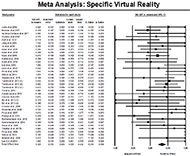
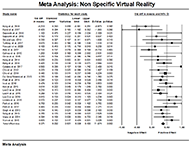
The results of the meta-analysis are presented in Figures 2 and 3. The Specific VR based intervention group included 37 individual studies that included a total of 1501 participants. The average range of effect sizes for the Specific VR based interventions were 0.547, ranging anywhere from -0.51 to 6.19. Of the 37 Specific VR based individual studies, 27 had a positive effect size whereas the other 10 had a negative effect size. The Specific VR based intervention groups received favorable results with a total effect size of 0.329, CI 0.226-0.432. This suggests a small to moderate effect of this VR intervention type on post-stroke UE motor rehabilitation. Alternatively, the Nonspecific VR based intervention groups included 29 individual studies with a total of 1102 participants and an average effect size range of 0.408 ranging from -0.42 to 1.2. Of the 29 Nonspecific VR based intervention studies only 3 studies had a negative effect size and the remaining 26 all had a positive effect size. The Nonspecific VR based interventions received slightly more favorable results with a greater total effect size of 0.371, CI 0.308-0.433. This effect size falls within the same small to moderate effect classification as the Specific VR based intervention group.
Quality analysis
The AMSTAR was used to evaluate the methodological quality of the reviews included in the meta-analysis. Studies rated to be high quality if scoring 8-11, moderate quality if scoring 4-7, or low quality if scoring is 0-3 [13]. According to our Risk of Bias Table analysis all 4 of the reviews received a score of 10-11 points, indicating these reviews are considered high quality based on the AMSTAR scale. One limitation that is evident from the risk of bias table is that half of the reviews (2 out of 4) did not include a list of both the included and excluded studies.
DISCUSSION
Summary of the results
Based on the assumption established by Maier and colleagues [9], specific VR systems are constructed with respect to evidence-based neurorehabilitation principles, which should induce greater motor recovery post stroke. These principles include: massed practice (repetitions), dosage (at least 5 hours per week), structured practice, task specific intervention, variable practice, multisensory interventions, varying difficulty, implicit/explicit feedback, and promoted use of the affected limb. However, the study conducted by Maier and colleagues [9] was the only study in this review that directly reported a greater effect for specific VR systems over nonspecific. The remaining studies incorporated in this review either only studied one type of VR system or incorporated both systems but did not differentiate between the two in their statistical comparisons. This further illustrates the reasoning behind our proposal to complete this meta-analysis.
The results of this meta-analysis found that there was virtually no difference between the type of VR system utilized. Both groups achieved the same small to moderate effect size after statistical comparison. These results demonstrate evidence that refutes the conventional assumption reported by Maier and colleagues [9]. One explanation that builds on the original assumption, which purports that specific VR systems include a greater number of neurorehabilitation principles, is that nonspecific VR systems indirectly apply neurorehabilitation principles by inducing greater client participation in therapy. Commercial gaming systems have become a well-known and easily identifiable form of technology within the general population. Clients may be more likely to buy into a treatment that utilizes these commercial gaming systems as a part of their rehabilitation process because these systems are typically associated as fun or enjoyable. Nonspecific VR systems are also, by definition, more readily available to consumers. Many clients may already have an appropriate system that they can utilize to participate in "therapy interventions" outside of the clinic setting. These factors help to facilitate increased dosage, repetition, and time spent using the affected limb. This aligns with the basis of effectiveness for other motor rehabilitation interventions such as Constraint Induced Movement Therapy [3]. The original assumption that specific VR systems incorporate a greater number of neurorehabilitation principles may be offset by the assumption that nonspecific VR systems facilitates a greater total time spent utilizing the affected limb, helping to explain why both systems achieved relatively equal effect sizes on motor recovery.
Implications for Research
Our findings suggest that regardless of the type of system used, VR based interventions are more effective than conventional therapy in facilitating upper extremity motor recovery in post stroke patients. Because no difference between VR systems was found, therapists considering implementing VR based interventions should select the system that best matches the factors surrounding the client, or the system that is more practical and accessible to incorporate in the rehabilitation setting.
Conclusion
Evidence from this meta-analysis suggests both specific and non-specific VR systems seem to be effective neurorehabilitative technological tools for improving UL motor function for individuals post-stroke. Our findings suggest that VR is well suited as a rehabilitative intervention since it allows the patient to participate in a safe and virtually accessible environment in which the intensity, repetition, and dosage of VR can all be controlled and modulated in a goal-oriented manner. Future studies should not question the efficacy of VR systems, but rather provide more insight on how to improve the methodology of future VR interventions.
REFERENCES
- Hatem, S. M., Saussez, G., Della Faille, M., Prist, V., Zhang, X., Dispa, D., & Bleyenheuft, Y. (2016). Rehabilitation of motor function after stroke: A multiple systematic review focused on techniques to stimulate upper extremity recovery. Frontiers in Human Neuroscience, 10, 442. https://doi.org/10.3389/fnhum.2016.00442
- Turolla, A., Dam, M., Ventura, L., Tonin, P., Agostini, M., Zucconi, C., Kiper, P., Cagnin, A., & Piron, L. (2013). Virtual reality for the rehabilitation of the upper limb motor function after stroke: A prospective controlled trial. Journal of NeuroEngineering and Rehabilitation, 10(85). https://doi.org/10.1186/1743-0003-10-85
- Kwakkel, G., Kollen, B. J., van der Grond, J., & Prevo, A. J. (2003) Probability of regaining dexterity in the flaccid upper limb: Impact of severity of paresis and time since onset in acute stroke. Stroke, 34(9), 2181-2186. https://doi.org/10.1161/01.STR.0000087172.16305.CD
- Saposnik, G., & Levin, M. (2011). Virtual reality in stroke rehabilitation: A meta-analysis and implications for clinicians. Stroke, 42(5), 1380-1386. https://doi.org/10.1161/STROKEAHA.110.605451
- Kleim, J. A., & Jones, T. A. (2008) Principles of experience-dependent neural plasticity: Implications for rehabilitation after brain damage. Journal of Speech, Language, and Hearing Research, 51(1), S225–239. https://doi.org/10.1044/1092-4388(2008/018)
- Langhorne, P., Coupar, F., & Pollock, A. (2009) Motor recovery after stroke: A systematic review. Lancet Neurology, 8(8), 741–754.
- Viñas-Diz, S., & Sobrido-Prieto, M. (2016). Virtual reality for therapeutic purposes in stroke: A systematic review. Neurologia, 31(4), 255–277. https://doi.org/10.1016/j.nrl.2015.06.012
- Shea, B. J., Grimshaw, J. M., Wells, G. A., Boers, M., Andersson, N., Hamel, C., Porter, A. C., Tugwell, P., Moher, D., & Bouter, L. M. (2007). Development of AMSTAR: A measurement tool to assess the methodological quality of systematic reviews. BMC Medical Research Methodology, 7(10). https://doi.org/10.1186/1471-2288-7-10
- Maier, M., Ballester, B. R., Duff, A., Oller, E. D., & Verschure, P. (2019). Effect of specific over nonspecific VR-based rehabilitation on poststroke motor recovery: A systematic meta-analysis. Neurorehabilitation and Neural Repair, 33(2), 112–129. https://doi.org/10.1177/1545968318820169
- Laver, K. E., Lange, B., George, S., Deutsch, J. E., Saposnik, G., & Crotty, M. (2017). Virtual reality for stroke rehabilitation. The Cochrane Database of Systematic Reviews, 11(11), CD008349. https://doi.org/10.1002/14651858.CD008349.pub4
- Lee, H. S., Park, Y. J., & Park, S. W. (2019). The effects of virtual reality training on function in chronic stroke patients: A systematic review and meta-analysis. BioMed Research International, 2019(1), Article 7595639. https://doi.org/10.1155/2019/7595639
- Domínguez-Téllez, P., Moral-Muñoz, J. A., Salazar, A., Casado-Fernández, E., & Lucena-Antón, D. (2020). Game-based virtual reality interventions to improve upper limb motor function and quality of life after stroke: Systematic review and meta-analysis. Games for Health Journal, 9(1), 1–10. https://doi.org/10.1089/g4h.2019.0043
- Lu, C., Lu, T., Ge, L., Yang, N., Yan, P., & Yang, K. (2020, May). Use of AMSTAR-2 in the methodological assessment of systematic reviews: Protocol for a methodological study. Annals of Translational Medicine, 8(10) 652. https://www.ncbi.nlm.nih.gov/pmc/articles/PMC7290613/.